Nature of chemical bonding in complex compounds
Structure physico-chemical and biological properties of complex compounds depend on the nature of chemical bonds in them. Currently, the nature of chemical bonds in complex compounds such theories explain:
1) the method of valence bonds ;
2) the crystal field theory ;
3) the method of molecular orbitals .
Let us consider the simpler method of valence bonds. Under this method, the connection between the central atom and the ligand is formed by the donor- acceptor mechanism. The donor is a ligand that donates a lone electron pair acceptor - Central heating atom, which has free orbitals.
For example, during the formation of the ion [Zn(NH3)4]2+ is a donor molecule of ammonia, in which the nitrogen atom has an unshared electron pair. Acceptor is a zinc atom, which has free d - orbitals. Electron- graphic formula of the third and fourth energy levels of zinc ions Zn+2 has the form:
Lone pairs of the nitrogen atoms of four ammonia molecules located on the ligand-free 4s-and 4p- orbitals of the zinc ion:
Properties of complex compounds
1) Dissociation of complex compounds.
Complex compounds are strong electrolytes. In aqueous solution, they readily dissociate to a complex ion and the outer sphere. This is called the primary dissociation. Examples of primary dissociation of complexes are given in Table
Dissociation of the complex compounds
Types of complex connections | Dissociation equation |
Cationic complex | [Ag(NH3)2]Cl ↔ [Ag(NH3)2]+ + Cl – [Cr(H2O)6]Cl3 ↔ [Cr(H2O)6]3+ + 3Cl – |
Anionic complex | K4[Fe(CN)6] ↔ 4K+ + [Fe(CN)6]4- Na2[Zn(OH)4] ↔ 2Na+ + [Zn(OH)4]2+ |
The formed complex ion may also dissociate, albeit weaker. This secondary dissociation, which is stepwise:
I step | [Ag(NH3)2]+ ↔ Ag(NH3)+ + NH3 |
II step | Ag(NH3)+ ↔ Ag+ + NH3 |
We can write the equation in a secondary dissociation step of:
[Ag(NH3)2]+ ↔ Ag+ + 2NH3
2) Stability of complex compounds. In general, chemical equilibrium is reached when the forward reaction rate is equal to the reverse reaction rate and can be described using an equilibrium constant, K. Complex ion equilibria are no exception to this and have their own unique equilibrium constant. This formation constant (Kf), describes the formation of a complex ion from its central ion and attached ligands. This constant may be called a stability constant or association constant; the units depend on the specific reaction it is describing.
|
|
At its most basic level, Kf can be explained as the following, where M is a metal ion, L is a ligand, and x and y are coefficients:
Kf values tend to be very large in magnitude because complex ion formation reactions heavily favor the product.
For example, observe the formation reaction of the dicyanoargentate (I) ion and the resulting formation constant expression:
The larger the Kf value of a complex ion, the more stable it is. Due to how large formation constants often are it is not uncommon to see them listed as logarithms in the form logKf. You may also see them in the form of a dissociation constant, Kd (which should not be confused with pKa). This is merely the inverse value of the formation constant and such describes the instability of a complex ion. It may help to think of Kf and Kd as stability and instability constants, respectively.
Using the above example, the dissociation constant expression would be:
In logarithm form Kf and Kd would be: logKf = log(5.6×1018) = 18.7
logKd = log(1.8×10−19) = −18.7
Today we will synthesize two of these compounds. One of them is a type of coordination compound called a chelate. In a chelate, the ligand has more than one point of attachment to the metal atom or ion. The ligand is called polydentate (“many toothed”). Three oxalate ions attach to an iron ion to give:
The oxalate ion, C2O42-, has two points of attachment to the iron ion, so it is bidentate. Three oxalate ions arrange themselves about the iron ion in an octahedral geometry. Note that the iron ion must have a 3+ charge in this substance in order to account for the 3– charge on the ion. The iron chelate will be crystallized out of solution as the potassium salt.
The other coordination compound you will prepare has a unidentate ligand, ammonia, attached to a copper ion in four positions:
The nitrogen in ammonia covalently bonds to the copper using its unbonded pair of electrons. Four ammonia molecules arrange themselves about the copper ion in a square planar geometry. The copper has a 2+ charge in this substance. The copper complex will be crystallized out of solution as the sulfate salt.
|
|
1st Experiment. Synthesis of Potassium tris(oxalato)ferrate (III)
The reaction is:
FeCl3 + 3K2C2O4 → K3[Fe(C2O4)3] + 3KCl
All of the substances are in aqueous solution. You will allow the green complex iron salt to precipitate by forming a mixed solvent. In this case, the mixed solvent is created by adding ethanol to a water solution of the iron complex. When the complex ion salt precipitates, it carries three molecules of water with it, so that the solid crystals have the formula: K3[Fe(C2O4)3]·3H2O.
Procedure:
While you are making the iron compound, you will also prepare a solution of copper sulfate that will be used in the 2nd experiment.
Set up a hot water bath.
Add water to the beaker to about the 150 ml mark. Heat the water in the beaker to boiling. Once it boils, reduce the flame to low heat so that the water simmers.
For Part 2 of the experiment, weigh out 1.0 gram of CuSO4*5H2O on a tared piece of paper. First fold the paper to put a crease in it, and then unfold it. The crease will help to pour the solid into a test tube. Add the solid to a test tube. Add 2.5 ml of deionized water to the test tube and place it in the hot water bath.
For Part 1, use the transfer pipets on the reagent bottles to add 1 ml of 1 M FeCl3 solution and 2 ml of a saturated K2C2O4 solution to the other test tube and place the test tube in the hot water bath. Leave it in the bath for 5 minutes.
Stir the contents of the copper sulfate test tube for Part 2 until all of the solid is dissolved, then remove the test tube from the bath and set it in the test tube rack.
Turn the bunsen burner off. Use the transfer pipet on the reagent bottle to add 1.5 ml of ethanol to the 50 ml glass beaker. Do not bring ethanol to your work station until the burner is off. Pour the hot, green contents of the test tube still in the hot water bath into the beaker and stir. Put the watch glass on the beaker and set the beaker out of the way. The watch glass goes on curved side down. Crystals should form while you work on Part 2. If no crystals appear within 15 minutes, add an inch of ice to a 250 ml beaker and set the 50 ml beaker on top of the ice. Rub the stirrer across the inside bottom of the beaker a few times. This can seed the solution with nanometer sized chunks of glass that often initiate crystal growth. The crystals should grow for at least 30 minutes.
|
|
While you are waiting, go on to Part 2 of the experiment.
Then decant the liquid from the crystals into the waste beaker. Add 2 ml of ethanol to rinse the crystals. Swirl, and decant the liquid from the rinse into the waste beaker. Use the rubber policeman to transfer the crystals onto a piece of filter paper setting on a double folded piece of paper towel. Spread the crystals out and allow to dry. Weigh the crystals on a piece of weighing paper. Put them in the container provided and keep them. Label the container with the formula and weight of the substance, as well as your name and the date. Use ink on white tape for the label. Show the sample to the instructor when you turn in your lab. Discard liquid from the waste beaker into the container in the fume hood marked “Iron Waste”.
The iron compound is light sensitive. Over time, if it is kept in the light, it will turn a rusty brown.
2nd Experiment. Synthesis of Tetramminecopper (II) sulfate
The compound which will be prepared in this experiment is tetraamminecopper (II) sulfate monohydrate, a coordination compound which is also a hydrate. A hydrate is a compound which contains loosely bound water (called water of hydration) in its structure. You can recognize the formula of a hydrate by the dot with a certain number of water molecules after which follows the formula for the anhydrous compound (the compound without the water. The equation for the overall reaction is: CuSO4 + 4NH3 + H2O --> [Cu(NH3)4]SO4 · H2O
The synthesis will be carried out in three steps:
1) The anhydrous copper (II) sulfate (CuSO4) will be dissolved in water:
|
|
CuSO4(s) ---> Cu2+(aq) + SO42-(aq)
2) The dissolved copper (II) ion (in step one) will be reacted with ammonia to form the complex ion [Cu(NH3)4]2+ :
Cu2+(aq) + 4NH3(aq) + SO42-(aq) ---> [Cu(NH3)4]2+(aq) + SO42-(aq)
3) The final product will be precipitated with ethanol in which it is insoluble:
ethanol
[Cu(NH3)4]2+(aq) + SO42-(aq) + H2O ---> [Cu(NH3)4]SO4 · H2O(s)
The reaction equation for step (2) indicates that one mole of Cu2+, which comes from one mole of CuSO4, reacts with four moles of NH3 (ammonia) to form one mole of the tetraamminecopper (II) ion. One mole of coordination compound is produced in step (3) from one mole of the tetraamminecopper (II) ion; therefore, in the overall reaction, one mole of coordination compound is obtained for every one mole of copper (II) sulfate reacted. The amount of ammonia required to react with any given amount of copper (II) sulfate can be calculated from the equation in step (2).
Safety Disposal Methods: Addition of ammonia should be performed under the hood. Disposal of the filtrate should be done in the drains under the hood with lots of water running. The precipitate should be disposed of in the labeled container under the hood.
PROCEDURE:
Дата добавления: 2018-09-20; просмотров: 599; Мы поможем в написании вашей работы! |
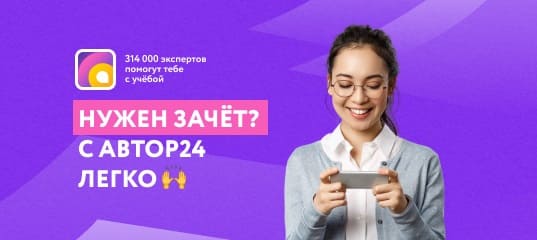
Мы поможем в написании ваших работ!