Drugs affecting blood vessels
Many different drugs and endogenous substances cause constriction or dilation of blood vessels. Drugs that have a direct relaxant effect on vascular smooth muscle include the organic nitrates (e.g., nitroglycerin tablets, which are mainly used to treat angina) and calcium antagonists (e.g., nifidipine). Most blood vessels are controlled by the sympathetic nervous system, and they constrict in response to norepinephrine released from sympathetic nerves. Thus, drugs that affect the sympathetic system cause constriction or dilation of blood vessels. The parasympathetic nervous system is much less important in controlling blood vessels.
Apart from the actions of the sympathetic nervous system, several other physiological mechanisms regulate vascular smooth muscle. Of particular pharmacological importance are the renin-angiotensin system and locally acting vasodilator substances, such as histamine, bradykinin, and prostaglandins.
Renin is an enzyme that is released into the bloodstream by the kidney when the blood pressure falls. It acts on a plasma protein to produce a peptide, angiotensin I, which consists of a chain of 10 amino acids. This in turn is acted on by angiotensin converting enzyme (ACE) to produce an eight-ammo acid peptide, angiotensin II (a potent vasoconstrictor), which raises the blood pressure. Inhibitors of ACE are used in treating high blood pressure.
Various substances that act on blood vessels are released when tissues are damaged by disease or injury. Histamine is stored by special cells in the skin and elsewhere, and when it is released histamine causes capillary walls to leak fluid, resulting in local tissue swelling. Prostaglandins and bradykinin have similar functions. All of these substances apparently act locally in the process of inflammation rather than systemically in overall cardiovascular regulation.
Inotropic agents
Inotropic agents are drugs that influence the force of contraction of cardiac muscle, thereby tending to affect the cardiac output. Drugs have a positive inotropic effect if they increase the force of contraction of the heart. The most important group of inotropic agents is the cardiac glycosides, substances that occur in the leaves of the foxglove (Digitalis purpurea) and other plants.
Although they have been used for many purposes throughout the centuries the effectiveness of cardiac glycosides in heart disease was established in 1785 by an English physician, William Withering who successfully used an extract of foxglove leaves to treat heart failure. Many closely related glycosides with similar pharmacological actions are found in various plants, but they differ in ease of absorption from the gastrointestinal tract and in duration of action. The two compounds most often used therapeutically are digoxin and digitoxin.
|
|
The most useful effect of cardiac glycosides is their ability to increase the force of contraction of cardiac muscle. They have, however, several additional effects, most of which are disadvantageous. These include a tendency to block conduction of the electrical impulse that causes contraction as it passes from the atria to the ventricles of the heart (heart block). Cardiac glycosides also have a tendency to produce an abnormal cardiac rhythm by causing electrical impulses to be generated at points in the heart other than the normal pacemaker region, the cells that rhythmically maintain the heartbeat These irregular impulses result in ectopic heartbeats that are out of sequence with the normal cardiac rhythm. Occasional ectopic beats are harmless, but if this process continues to a complete disorganization of the cardiac rhythm (ventricular fibrillation), the pumping action of the heart is stopped, causing death within minutes unless resuscitation is carried out. Because the margin of safety between the therapeutic and the toxic doses of glycosides is relatively narrow, they must be used carefully.
Cardiac glycosides are believed to increase the force of cardiac muscle contraction by binding to and inhibiting the action of a membrane enzyme that extrudes sodium ions from the cell interior. Inhibiting the free flow of sodium ions from the interior of the cell across the membrane to the exterior of the cell causes the intracellular sodium concentration to rise. The interior of the cell then becomes depolarized, or electrically less negative than normal with respect to the exterior of the cell. Because the cell is able to exchange sodium ions within the cell for calcium ions outside it, there is a secondary rise in intracellular calcium. This subsequently increases the force of contraction, since intracellular calcium ions are responsible for initiating the shortening of muscle cells.
|
|
The disturbances of rhythm that may be caused by cardiac glycosides result partly from the depolarization and partly from the increase in intracellular calcium. Because these rhythm disturbances are caused by the same underlying mechanism that causes the beneficial effect, there is no likelihood of finding a cardiac glycoside with a significantly better margin of safety. Apart from their cardiac actions, these glycosides tend to cause nausea and loss of appetite. Because digoxin and digitoxin have long plasma half-lives (two and seven days, respectively), they are liable to accumulate in me body. Treatment with either of these drugs must involve careful monitoring to avoid the adverse effects that may result from their slow buildup in the body.
The second type of inotropic agent that increases the force of cardiac muscle contraction includes epinephrine and norepinephrine. In addition to affecting the force of contraction, however, they also increase the heart rate. This, and the fact that they are quickly metabolized by the body and act only for a few minutes, means that they are not useful inotropic agents.
The third type of inotropic agent that acts as a cardiac stimulant is the caffeine-related series of drugs represented by theophylline.
The heart rate is controlled by the opposing actions of sympathetic and parasympathetic nerves and by the action of epinephrine released from the adrenal gland. Norepinephrine, released by sympathetic nerves in the heart, and epinephrine, released by the adrenal gland, increase the heart rate, while acetylcholine, released from parasympathetic nerves, decreases it. A competitive antagonist that acts to inhibit the stimulating action of norepinephrine on the heart is propranolol, which slows the heart and is often used to treat anginal attacks and disturbances of cardiac rhythm. Atropine blocks acetylcholine receptors and is used during anesthesia to prevent excessive cardiac slowing.
Anticoagulant drugs
Anticoagulant drugs prevent the formation of thrombi by inhibiting the coagulation phase. They are used to prevent the formation and spread of venous and arterial thrombi; however, they are ineffective against existing thrombi. Anticoagulant therapy is used to treat deep-vein thrombosis and pulmonary embolism arising after immobilization or surgery; systemic or coronary arterial embolism caused by heart diseases or replacement of the prosthetic valve;and disseminated intravascular coagulation, which is a systemic activation of the system that leads to consumption of coagulation factors and hemorrhage.
|
|
Heparin, used primarily in hospitalized patients, is a mixture of negatively charged mucopolysaccharides. An endogenous substance whose physiological role is not understood, heparin blocks the coagulation cascade by promoting the interaction of a circulating inhibitor of thrombin (antithrombin III) with activated clotting factors. Because it is not well-absorbed when taken orally, heparin is given intravenously to inhibit coagulation immediately; the onset of the drug's effect is delayed after subcutaneous administration. Heparin is not bound to plasma proteins, it is not secreted into breast milk, and it does not cross the placenta. The drug's action is terminated by metabolism in the liver and excretion by the kidney. The major side effect associated with heparin is hemorrhage; thrombocytopenia (reduced number of circulating platelets) and hypersensitivity reactions also occur. Oral anticoagulants and heparin have additional anticoagulant effects. Heparin-induced hemorrhage may be reversed with the antagonist protamine, a positively charged protein that has a high affinity for heparin's negatively charged molecules, thus neutralizing the drug's anticoagulant effect. When given in combination with heparin, dihydroergotamine, which constricts veins and increases blood flow, increases heparin's antithrombotic effect.
Oral anticoagulants are derivatives of 4-hydroxycoumarin (coumarin) or indan-l,3-dione (indandione). Structurally the coumarin derivatives resemble vitamin K, an important element in the synthesis of a number of clotting factors. Interference in the metabolism of vitamin K in the liver by coumarin derivatives gives rise to clotting factors that are defective and incapable of binding calcium ions (another important element in the activation of coagulation factors at several steps in the coagulation cascade). When anticoagulants are taken orally, several hours are required for the onset of the anticoagulant effect because time is required both for their absorption from the gastrointestinal tract and for the clearance of biologically active clotting factors from the blood. Warfarin, the most commonly used oral anticoagulant, is rapidly and almost completely absorbed; the absorption of dicumarol and other anticoagulant agents, however, is slower and less consistent.
|
|
Oral anticoagulants bind extensively to plasma proteins, have relatively long plasma half-lives, and are metabolized by the liver and excreted in the urine and feces. They may cross the placenta to cause fetal abnormalities or hemorrhages in neonates; their appearance in breast milk apparently has no adverse effect on nursing infants. Hemorrhage is the principal toxic effect during oral anticoagulant therapy, but each of the coumarin derivatives causes its own idiosyncratic side effects. Vitamin K, when given intravenously to promote the synthesis of functional clotting factors, stops bleeding after several hours. Plasma that contains normal clotting factors is given to control serious bleeding. Oral anticoagulants may interact adversely with other drugs that bind to plasma proteins or are metabolized by the liver.
Fibrinolitic drugs
When a small blood vessel is cut, a repair mechanism (hemostasis) is activated that eventually seals the cut and prevents further blood loss. What is in fact a lifesaving mechanism that protects the wounded body from hemorrhage becomes life threatening when clots (thrombi) form within functional blood vessels (thrombosis). Thrombosis tends to occur in blood vessels damaged by artherosclerosis or in vessels with a sluggish blood flow. In veins, portions of the thrombi (emboli) may break off and pass along the bloodstream to become lodged in the arteries of the heart. The drugs described in this section either inhibit hemostasis or they act to enhance the mechanisms that lyse, or dissolve, trombi.
The clotting process essentially involves the conversion of a soluble plasma protein, fibrinogen, into strands of the insoluble protein fibrin, which forms a mesh that traps platelets. The trigger for hemostasis is an injury to the endothelium, the cells lining the blood vessels, so that the underlying layer of collagen is exposed. The series of events leading to clot formation in a cut blood vessel are (1) constriction of the blood vessel by serotonin, epinephrine, and the thromboxane A, which diminishes blood loss; (2) formation of a plug of platelets (the platelet phase) by ADP and thromboxane A, also released by platelets, which act in a positive feedback process that makes more platelets adhere to the collagen and to each other, and (3) the conversion of the plug into a clot of fibrin (the coagulation phase). The formation of fibrin entails the sequential interaction of more than a dozen clotting factors, which are protease enzymes (i.e., they accelerate the breakdown of proteins). Each of these clotting factors activates the next in a coagulation cascade of proteolytic reactions that break down protein molecules. The penultimate reaction is the conversion of the soluble fibrinogen to soluble fibrin under the influence of the enzyme thrombin (factor IIa). Soluble fibrin is converted to insoluble fibrin strands by activated factor XIII (fibrin-stabilizing factor), and covalent cross-linkages form between the fibrin strands to give a strong and rigid network. Several of the clotting factors (II. VII, IX, X) require the presence of vitamin K for their activation. Consequently, inhibition of vitamin K blocks the propagation of coagulation pathways.
Under normal conditions the adhesion of platelets to vessel walls is prevented by the vascular endothelial cells, at least in part by their ability to release prostaglandins called prostacyclin or prostaglandin I, which reduce platelet stickiness and cause dilation of the blood vessels.
A fibrinolytic system exists in the body that restricts thrombus propagation beyond the site of injury and is also involved in the lysis of clots as wounds heal.
Fibrinolytic drugs activate the fibrinolytic pathway and lyse clots. The fibrinolytic drugs are distinct from the coumarin derivatives and heparin, which inhibit the formation of clots.
Tissue plasminogen activator (TPA) stimulates fibrinolysis, and it has several important advantages over streptokinase and urokinase in treating coronary thrombosis. It binds readily to fibrin and after intravenous administration activates only the plasminogen that is bound to the thrombus; thus, fibrinolysis occurs in the absence of an extensive breakdown of the coagulation factors. It may be used to initiate treatment of heart attack victims en route to the hospital, eliminating the time spent in the hospital preparing the patient for intracoronory injections of sfreptokinase. This is extremely useful because the rapid reestablishment of coronary blood flow is critically important to minimize the amount of damage to myocardial cells after an infarction.
An elevation in the level of circulating plasmin because of excessive activation of the fibrinolytic system may result in fibrinogenolysis and hemorrhage. The antifibrinolytic drug aminocaproic acid is a specific antagonist of plasmin and inhibits the effects of fibrinolytic drugs.
Streptokinase is produced from streptococcal bacteria. When administered systemically, streptokinase lyses acute deep-vein, pulmonary, and arterial thrombi; however, the drug is less effective in treating chronic occlusions. Streptokinase administered by intracoronary artery infusion soon after a coronary occlusion has formed is effective in reestablishing the flow of blood through the heart and vessels after a myocardial infarction and in limiting the size of the area of infarct (or tissue death). Intracoronary infusion permits the delivery of a high concentration of the drug to a localized area and speeds the activation of the fibrinolytic pathway. Intracoronary infusion minimizes the amount of streptokinase inactivated by antibodies that are normally present in blood. Heparin and aspirin can be added to therapy to help prevent the recurrence of occlusive thrombi. An overdose of streptokinase may lead to bleeding from systemic fibrinogenolysis, which is the breakdown of the coagulation factors by plasmin.
Urokinase is a protease enzyme that activates plasminogen directly. Because it is obtained from tissue culture of human kidney cells, it is not antigenic. Urokinase lyses recently formed pulmonary emboli, and, compared to streptokinase, it produces fibrinolysis without extensive breakdown of the coagulation factors. The usefulness of intravenous or intracoronary urokinase after myocardial infarction is not known.
Analgesics and narcotics
Analgesics are drugs that relieve pain selectively without affecting consciousness or sensory perception. This selectivity in relieving pain is the important distinction between an analgesic and an anesthetic. Analgesics are often self-prescribed and abused. Many strong analgesics can produce serious and even fatal depression of the central nervous system. Furthermore, the use of analgesics can involve such problems as addiction, fatal overdose, allergic reaction, and significant gastrointestinal irritation. Analgesics may be classified into two types: the opioids, which act on the brain; and anti-inflammatory drugs, which alleviate pain by reducing local inflammatory responses.
The opioid analgesics were once called narcotic drugs because they induce sleep and cause dependence or addiction. This term is no longer used by physicians or scientists since both dependence and addiction can be induced by many drugs other than opioids. The opioid analgesics can be used for either short-term or long-term relief of severe pain. In contrast, the anti-inflammatory compounds are non-narcotic analgesics and are used for short-term pain relief and for modest pain, such as that of headache, muscle strain, bruises, or arthritis.
The term opioid has been adopted as a general classification of all of those agents that share chemical structures, sites, and mechanisms of action with the endogenous opioid agonists. Opioid substances encompass all of the natural and synthetic chemical compounds closely related to morphine, whether they act as agonists or antagonists. Although interest in these drugs has always been high because of their value in pain relief and because of problems of abuse and addiction, interest was intensified in the 1970s and 1980s by discoveries about the naturally occurring morphine-like substances, the endogenous opioid neuropeptides.
Opioid drugs are useful in the treatment of general postoperative pain, severe pain, and other specific conditions. The use of opiates to relieve the pain associated with kidney stones or gallstones presumably depends on their ability to affect opiate receptors in these tissues and to inhibit contractility. By a similar mechanism, opiates are also able to relieve the abdominal distress and fluid loss of diarrhea. Central receptors appear to account for the ability of morhine and analogues to suppress coughing, an effect that requires lower doses than those needed for analgesia. Low doses of opiates given subcutaneously are also specifically advocated for the relief of the respiratory distress that accompanies acute cardiac insufficiency complicated by the buildup of fluid inthe lungs, even though the mechanisms of this effect are unknown and despite the fact that opiates are respiratory depressants.
The effectiveness of a given dose of an opiate drug declines with its repeated administration in the presence of intense pain. This loss in effectiveness is termed tolerance. Evidence suggests that tolerance is not due to alterations in the brain's responses to drugs. Animals exhibiting tolerance to morphine after repeated infections in a familiar environment show little or no tolerance when given the same doses and tested for pain sensitivity in new environments. Thus, a learned aspect of tolerance seems almost certain. The cellular and molecular mechanisms underlying this loss of responsiveness are not clear, however. Physical dependence and addiction in a person using intravenous administration closely follow the dynamics of drug tolerance; increasing doses are required to produce the psychological effects, while tolerance protects the brain against these respiratory depressant actions of the drug. In the tolerant individual, intense adverse reactions can be precipitated by administration of an opiate antagonist, thus revealing the dynamic internal equilibrium that previously appeared to neutralize the response of the brain to the opiates. The signs of the withdrawal response (yawning, tearing, perspiration, dilation of me pupils, nasal discharge, anxiety, tremors, elevation of blood pressure, abdominal cramps, and hyperthermia) can be viewed as signs of an activated sympathetic nervous system and to some extent an extreme, but nonspecific, arousal response. This may also suggest that tolerance in the face of increasing opiate doses is more properly viewed as a balancing mechanism by this sympathetic activation.
Anti-inflammatory analgesics
Several chemically unrelated series of complex organic acids have the ability to relieve mild to moderate pain through actions that reduce inflammation at its source. The prototype of this class of drugs is acetylsalicylic acid, or aspirin. Like aspirin, many of the drugs of this class also reduce fever (antipyretic action), and those that resemble aspirin most closely share what is presumed to be its molecular mechanism of action, namely inhibition of the synthesis of prostaglandins (natural products of inflamed leukocytes, which induce the responses in local tissue that include pain and inflammation). These three properties of anti-inflammatory drugs (i.e., analgesia, anti-inflammation, and antipyretic action) vary among the drugs.
Aspirin, which is now made by total chemical synthesis, is the most widely used mild analgesic, although more potent antipyretic analgesics, such as acetaminophen, are available. Super-aspirins, such as indomethacin, were developed in the 1970s as the mechanisms of critical analgesic action, namely prostaglandin synthesis inhibition, came to be understood.
Research has shown that small doses of certain prostaglandins can mimic almost all of the signs and symptoms of localized inflammation. Prostaglandins are naturally occurring by-products of arachidonic acid synthesis. They are thought to be released at the site of inflammation when leukocytes are attracted to injured or inflamed areas. All mammalian cells except red blood cells can produce prostaglandins, and when injured, the cells release large amounts of these substances. All aspirin-like analgesics inhibit prostaglandin synthesis and release, and their varying potencies depend on the degree to which they can do so.
As might be expected from their common mechanisms of action, many of the non-narcotic analgesic drugs share similar side effects. Hypersensitivity responses to aspirin-like drugs are thought to be due to an accumulation of prostaglandins after the pathways that break down prostaglandins are blocked. These responses can be fatal when very strong anti-inflammatory compounds are given. Inhibition of prostaglandin synthesis may result in other serious side effects, such as gastric ulcers (which may also be due in part to the irritant activity of large doses of aspirin on the lining of the stomach) and the reduced ability of platelets in the blood to aggregate and form clots. The latter effect however, has given aspirin an added use as a prophylactic antithrombotic drug to reduce chances of cardiac or cerebral vascular thrombosis. Some of these aspirin-like analgesics also have specific toxic effects: liver damage occasionally occurs after administration of acetaminophen, and renal toxicity and behavioral symptoms are sometimes seen with use of the super-aspirin anti-inflammatory drugs. Aspirin is thought to be a causative agent of Reye's syndrome, a rare and serious degenerative disease of the brain and fatty tissue of the liver that accompanies certain viral infections in children and young adults.
For treatment of mild pain, two types of analgesics can be used as alternatives to aspirin. The first type comprises the para-aminophenol derivatives such as acetaminophen, which is not a particularly useful anti-inflammatory drug. The term nonsteroidal anti-inflammatory agents is generally reserved for the second type of aspirin-like analgesics, the newer class of highly potent compounds sometimes termed super-aspirins. All these drugs inhibit prostaglandin synthesis. The first of this series, indomethacin, has been largely discarded because of side effects. The most widely used of the super-aspirins are the proprionic acid derivatives, such as ibuprofen, naproxen, and fenoprofen. Although these drugs are widely tolerated and can give significant analgesia in moderate doses, they have also been shown to cause all of the side effects of the other inhibitors of prostaglandin synthesis.
Дата добавления: 2018-02-15; просмотров: 557; Мы поможем в написании вашей работы! |
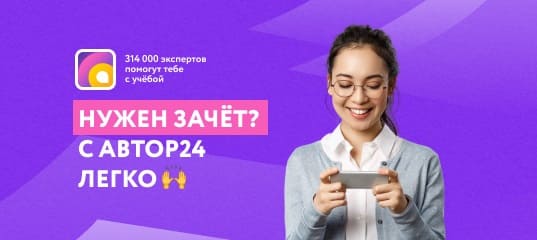
Мы поможем в написании ваших работ!