Simple hydrocarbons and their Variations
Nbsp; OIL An oil is any substance that is in a viscous liquid state ("oily") at ambient temperatures or slightly warmer, and is both hydrophobic (immiscible with water, literally "water fearing") and lipophilic (miscible with other oils, literally "fat loving"). This general definition includes compound classes with otherwise unrelated chemical structures, properties, and uses, including vegetable oils, petrochemical oils, and volatile essential oils. Oil is a nonpolar substance.
Biological
- Vegetable oils, non-volatile oils extracted from plants, usually from the seeds. Vegetable oils are less stable than mineral oils, and degrade over time.
- Essential oils, volatile aromatic oil extracted from plants.
- Animal Oils, such as whale oil and cod liver oil.
Synthetic
- Synthetic oils, synthesised
Mineral
-
Crude oil, petroleum, and many petroleum derivatives fuel oils.
Crude Oil
Crude oil is a complex mixture of hydrocarbons with minor proportions of other chemicals such as compounds of sulphur, nitrogen and oxygen. To use the different parts of the mixture they must be separated from each other. This separation is called refining.
Crude oils from different parts of the world, or even from different depths in the same oilfield, contain different mixtures of hydrocarbons and other compounds. This is why they vary from light coloured volatile liquids to thick, dark oils - so viscous that they are difficult to pump from the ground.
Physical and Chemical Properties of Oil
Oil is described by the physical properties of density, colour, viscosity, thermal expansion and other properties related to the number of carbon atoms in the molecules such as boiling point and composition.
COLOUR: depending on the additives and the conditions of formation, petroleum can be of different colours. Oil colours vary in a very wide range from oilfield to oilfield: from pale yellow, yellow and even colourless to dark grey, green and dark brown shades.
DENSITY: is the absolute relationship between mass and volume at a stated temperature. The SI unit is kg\ m3 at a reference temperature, typically 150C. Knowledge of density is required for quantity calculations. Specific gravity of a substance is the ratio of the mass of a given volume to the mass of an equal volume of water at the same temperature. As it is a ratio, there are no units. In the USA and some other countries the density of petroleum products is defined in terms of API gravity. This is an arbitary scale adopted by the American Petroleum Institute for expressing the relative density of oils. Density of oils range from 0.65 to 1.0 gr\cm3 and more at 200C. According to density, oils may be light, medium and heavy. Light oil is characterized by the density of 0.5 – 0.87; medium oil: 0.871 – 0.910 and heavy oil is described being as 0.910 – 1.05 gr\cm3. Oil specific density depends on the hydrocarbon types, the content of dissolved gases and gummy impurities and significantly varies with changing in its temperature (at increasing in temperature oil specific density decreases).
|
|
API gravity as it has been mentioned is a numeric scale based on density. Oil is made up of many different molecules of which the lighter is the more desirable one. Oil with the lighter molecules can be converted at least cost into gasoline and other valuable commodities. This refining conversion is accomplished through a process called cracking. In this process, a refinery separates the lighter molecules from the heavier ones, as well as, breaks apart the larger ones into small ones. Heavy weight oil is more expensive, therefore, the less dense the oil is – the lighter the oil and the more valuable it is. The API gravity scale is read “backwards”. The higher the API number, expressed as degrees API, the less dense (lighter, thinner) the oil is. Conversely, the lower the degrees API, the more dense (heavier, thicker) is the oil. Crude oils from different fields and from different formations within a field can be similar in composition or be significantly different. These differences in composition are very important to chemical engineers running a refinery. For crude oils (crudes) that have undergone detailed physical and chemical property analysis, the API gravity can be used as a rough index of the quality of the crudes with similar composition as they naturally occur (that is without adulteration, mixing, blending). When crudes of different types and quality are mixed or when different petroleum components are mixed, API gravity cannot be used meaningfully for anything other than a measure of the density of the fluid.
|
|
VISCOSITY: is a property of fluids that indicates their resistance to flow, defined as ratio of shear stress to shear rate. Crude oils range in consistency from water to tar-like solids. Viscosity may be visualized as a result of physical interaction of molecules when subjected to flow. Different fluids deform at different rates and different stress. Fluid with a high viscosity such as syrup deforms more slowly than fluid with a low viscosity such as water. Absolute viscosity is measured in poise (η = dyne*sec\cm3). When researching oil specific viscosity is usually defined as ratio of absolute viscosity of a given fluid η to absolute viscosity of water ηo at the same temperature. The viscosity of oil is dependent upon temperature, pressure and shear rate. Viscosity decreases at temperature increases because molecules vibrate and interact less. Conversely, the viscosity of oil increases at temperature decreases and it can become grease-like at very low temperature.
THERMAL EXPANSION: The volume of given oil mass increases with temperature, therefore, its density decreases. The degree of expansion is expressed as the coefficient of thermal expansion. Thermal expansion is useful to determine the size of container needed when the oil is heated. The coefficient of thermal expansion is the relative change of volume to a change in temperature. Thermal expansion is expressed as the ratio of volume change to initial volume after heating 10C. Thermal expansion (contraction) determinations require measurements of the volume of a given mass of oil at various temperatures.
BASE OF OIL COMPOSITION: Crude oils are complex mixtures containing hundreds of different hydrocarbon compounds (hydrocarbons) that vary in appearance and composition from oil field to oil field, therefore, in various oil fields the oil composition can vary significantly. Hydrocarbons are naturally occurring organic compound comprising hydrogen and carbon. They differ from each other in internal chemical arrangement and therefore in their chemical properties. All hydrocarbons are divided into two groups: saturated hydrocarbons and unsaturated hydrocarbons. Saturated hydrocarbons are not capable of attaching atoms and molecules while unsaturated hydrocarbons are capable of attaching atoms and molecules. The latter take part in chemical reactions easier. Hydrocarbons can be as simple as methane, but many are highly complex molecules and can occur as gases, liquids or solids. The molecules can have the shape of chains, branching chains, rings or other structures. An “average” crude oil contains about 84% carbon, 14% hydrogen, 1-5% sulfur, and less than1% of nitrogen, oxygen, metals and salts. Crude oils contain hundreds of different hydrocarbons and relatively small quantities of other organic and inorganic substances, which elements in addition to H and C are sulfur (S), nitrogen (N), and oxygen (O), as well as, metals – iron (Fe), vanadium (V), nickel (Ni), chromium (Cr), and others. Collectively, these substances are called heterocompounds. Crude oils contain salt and water. In the refinery, most of these non-hydrocarbon substances are removed and the oil is broken down into various compounds and blended into useful products.
|
|
OIL | |
Green oil | Зеленая нефть (нефть из шотландских сланцев) |
Black oil | Мазут; тяжелые нефтяные остатки |
Brown oil | Выветрившаяся нефть |
Hot oil | Нефть добывая из скважин сверх установленной нормы; незаконно добытая нефть |
Light oil | Легкая нефть (легкие фракции нефти); дизельное топливо |
Heavy oil | Тяжелая нефть |
Raw oil | Неочищенная нефть (сырая) |
Residual oil | Остаточная нефть |
Postulated oil | Предполагаемая нефть |
Rock oil | Пластовая нефть |
Second-tier oil | Новая нефть, не попадающая под ограничение цен в соответствии с законом США о сохранении энергии 1975 г. (старая нефть first - tier oil должна продаваться по фиксированным ценам) |
Sediment oil | Скопившаяся на дне хранилища нефтеводяная эмульсия с осадками, непригодная к эксплуатации в обычном порядке |
Shale oil | Сланцевое масло; продукты тепловой перегонки сланцев |
Skunk oil | Сильно пахнувшая нефть (вводимая в газопровод для обнаружения места утечки газа) |
Slashing oil | Масло, предохраняющее от ржавчины |
Stock oil (stock-tank) | Товарная нефть, приведенная к нормальным условиям |
Tar oil | Гудрон |
Tarry oil | Тягучая смолистая нефть |
Thinned oil | Газированная нефть |
|
|
HYDROCARBONS
What are Hydrocarbons?
Hydrocarbons vary in structure depending on the number of carbon atoms and the way in which the hydrogen atoms combine with them. Hydrocarbons can be arranged as straight chains, branch chains or closed rings. There are two main chemical families of hydrocarbons - the alkanes and the alkenes.
As the structure of hydrocarbons varies so much, thousands of synthetic products can be manufactured with many different properties. Hydrocarbons with small molecules make good fuels Methane (CH4) has the smallest molecules, and is a gas, used for cooking and heating and generating electricity. Gasoline, diesel, aviation fuel and fuel oil are all liquid fuels.
Hydrocarbon molecules can be split up into smaller ones, or built up into bigger ones, or altered in shape, or modified by adding other atoms. This is why they are a very useful starting point (called a chemical feedstock) for making other materials. Even the thick black tarry residue left after distillation is useful. It is called bitumen, and is used in tarmac for road surfacing, and for roofing.
In organic chemistry, a hydrocarbon is an organic compound consisting entirely of hydrogen and carbon. With relation to chemical terminology, aromatic hydrocarbons or arenes, alkanes, alkenes and alkyne-based compounds composed entirely of carbon or hydrogen are referred to as "Pure" hydrocarbons, whereas other hydrocarbons with bonded compounds or impurities of sulphur or nitrogen, are referred to as "impure", and remain somewhat erroneously referred to as hydrocarbons.
Hydrocarbons are referred to as consisting of a "backbone" or "skeleton" composed entirely of carbon and hydrogen and other bonded compounds, and lack a functional group that generally facilitates combustion without adverse effects. The majority of hydrocarbons found naturally occur in crude oil, where decomposed organic matter provides an abundance of carbon and hydrogen which, when bonded can catenate to form seemingly limitless chains.
Types of hydrocarbons
The following classifications for hydrocarbons defined by IUPAC nomenclature of organic chemistry are as follows:
1. Saturated hydrocarbons (alkanes) are the most simple of the hydrocarbon species and are composed entirely of single bonds and are saturated with hydrogen; they are the basis of petroleum fuels and are either found as linear or branched species of unlimited number. The general formula for saturated hydrocarbons is CnH2n + 2 (assuming non-cyclic structures).
2. Unsaturated hydrocarbons have one or more double or triple bonds between carbon atoms. Those with one double bond are called alkenes, with the formula CnH2n (assuming non-cyclic structures). Those containing triple bonds are called alkynes.
3. Cycloalkanes are hydrocarbons containing one or more carbon rings to which hydrogen atoms are attached. The general formula for a saturated hydrocarbon containing one ring is CnH2n
4. Aromatic hydrocarbons, also known as arenes which have at least one aromatic ring
Hydrocarbons can be gases (e.g. methane and propane), liquids (e.g. hexane and benzene), waxes or low melting solids (e.g. parafin wax and naphthalene) or polymers (e.g. polyethylene, polypropylene and polystyrene}.
General properties
Because of differences in molecular structure, the empirical formula remains different between hydrocarbons; in linear, or "straight-run" alkanes, alkenes and alkynes, the amount of bonded hydrogen lessens in alkenes and alkynes due to the "self-bonding" or catenation of carbon preventing entire saturation of the hydrocarbon by the formation of double or triple bonds.
This inherent ability of hydrocarbons to bond to themselves is referred to as catenation, and allows hydrocarbon to form more complex molecules, such as cyclohexane, and in rarer cases, arenes such as benzene. This ability comes from the fact that bond character between carbon atoms is entirely non-polar, in that the distribution of electrons between the two elements is somewhat even due to the same electronegativity values of the elements (~0.30), and does not result in the formation of an electrophile.
Generally, with catenation comes the loss of the total amount of bonded hydrocarbons and an increase in the amount of energy required for bond cleavage due to strain exerted upon the molecule; in molecules such as cyclohexane, this is referred to as ring strain, and occurs due to the "destabilized" spatial electron configuration of the atom.
In simple chemistry, as per valence bond theory, the carbon atom must follow the "4-hydrogen rule", which states that the maximum number of atoms available to bond with carbon is equal to the number of electrons that are attracted into the outer shell of carbon. In terms of shells, carbon consists of an incomplete outer shell, which comprises 4 electrons, and thus has 4 electrons available for covalent or dative bonding.
Simple hydrocarbons and their Variations
Number of carbon atoms | Alkane | Alkene | Alkyne | Cycloalkane |
1 | Methane | — | — | — |
2 | Ethane | Ethene | Ethyne | — |
3 | Propane | Propene | Propyne | Cyclopropane |
4 | Butane | Butene | Butyne | Cyclobutane |
5 | Pentane | Pentene | Pentyne | Cyclopentane |
6 | Hexane | Hexene | Hexyne | Cyclohexane |
7 | Heptane | Heptene | Heptyne | Cycloheptane |
8 | Octane | Octene | Octyne | Cyclooctane |
9 | Nonane | Nonene | Nonyne | Cyclononane |
10 | Decane | Decene | Decyne | Cyclodecane |
Oil Formation
Petroleum (literally rock oil, from the Greek petra- for rock and Latin -oleum for oil) is a general term used to refer to all forms of oil and natural gas that is mined from the earth. What most people concern themselves with is crude oil, the liquid mixture of naturally occurring hydrocarbons, and natural gas, which is a gaseous mixture of naturally occurring hydrocarbons. Hydrocarbons are complex molecules that are formed from long strings of hydrogen and carbon, such as propane (C3H8) or butane (C4H10).
Petroleum is the final product that we get out of the ground. But how does it get there? Petroleum begins as living animals, microscopic organisms (like diatoms or plankton) that live in the oceans. When these organisms die, their bodies sink and collect on the ocean floor. These organisms live all over the oceans and their bodies fall and collect on the ocean bottoms all over the world. When the organic matter becomes buried and begin to decompose, they are referred to as kerogen. Despite the apparent abundance of dead organisms raining down on the ocean bottoms, there are specific conditions that must be met for these organisms to be transformed into petroleum.
First, the area that the kerogen collects must be a restricted basin, a depression where sediment can accumulate and where there is poor water circulation. Here, the organisms will partially decompose and use up what little oxygen is available. When the oxygen is gone, the decomposition stops and the remaining matter are preserved. The kerogen must be buried under sediment where it will be altered through high temperatures and high pressures. As the heat and pressure breaks down the kerogen, the hydrocarbon chains are freed. Long chains of hydrocarbon are oil; shorter chains are gas, generally methane (CH4) and condensates such as ethane, propane and butane. As the heat and pressure continues, the longer chains will continue to break into shorter chains. If the process continues long enough, all that will remain will be methane.
Compaction of the sediment, and the expansion of the kerogen as it is transformed into petroleum cause it to be forced out of the rock it was created in ( source rock) and into nearby sediments. If these sediments are porous enough (have microscopic holes) and permeable enough (allowing the flow of liquids), then the petroleum will migrate through the rock. Since gas and oil are lighter than water, they can travel through water-saturated rock. Eventually the oil will stop migrating as it meets rock that is not porous or permeable, and will collect in a trap.
The process seems simple enough, millions of organisms die and are buried and become oil overtime, and it then migrates through the rock to places where it is trapped and the geologists can find it. Despite the simplicity, there are several conditions that must occur, otherwise, no oil will be made. First, there needs to be a source rock that contains the organic matter to be converted into petroleum. This source rock is generally shale or other mudstones. There must be a reservoir rock, usually sandstone or limestone that is porous and permeable where the oil can be stored and transported. There needs to be a trap, something that is non-porous and non-permeable that will hold the petroleum in the reservoir and prevent it from migrating further. Finally, there needs to be enough heat and pressure to sufficiently cook the oil and gas out of the kerogen. If any of these conditions is not met, then petroleum cannot be formed.
The important step in the process is the trap. Something needs to block or trap the petroleum so it will accumulate into a large enough deposit for geologists to be able to locate it. Petroleum traps come in several varieties, in various sizes and can be made through structural processes (like folds and faults), or by sedimentary processes.
Structural traps work by folding or breaking the reservoir rock and placing it adjacent to an impermeable rock layer, like shale. One of the most common is a trap from the folding of the rocks. Anticlines (see my article on folded rocks) bend the reservoir rock and create a pocket at the apex of the fold where the petroleum cannot migrate. Normal and thrust faults can result in petroleum traps by breaking the reservoir rock and moving it so that it is against an impermeable rock layer. The geologist who is looking for oil or gas in structurally complex regions needs a good understanding of structural geology.
The other way to trap petroleum is through stratigraphic traps. The differences between these and structural traps is that these traps occur by the nature of how the sediment was deposited and not whether it was broken or folded. Sandstone lenses and sandstone pinch-outs are the result of the changes in deposition of the sediment. Thick layers of mud are covered by thinner layers of sand from migrating shoreline, or by the sand deposited by large rivers. As sea level changes, or rivers migrate, the different sand and mud layers are interwoven creating lenses or pinch-outs. These sand layers allow the petroleum to accumulate and the mudrock layers trap the petroleum. Unconformities can create traps by burying truncated sandstone or limestone layers with layers of mudstone. Coral reefs are great places to trap oil. The open cavities between the corals create excellent reservoirs, and when the reef is buried by mud, the oil becomes trapped. Finally, salt domes can push up through buried sediment and deform the overlying layers of rock. This causes folds and fractures to form in the rock, trapping the oil
Fig. Evolution of kerogen in petroleum formation(from Tissot, 1984)
Legend: Principal products of kerogen evolution
![]() |
CO2 H2O
Oil
Gas
![]() |
Residual organic matter (no potential for oil or gas)
Trapping Oil and Gas
Oilfields and gasfields are areas where hydrocarbons have become trapped in permeable reservoir rocks, such as porous sandstone or fractured limestone. Migration towards the surface is stopped or slowed down by impermeable rocks such as clays, cemented sandstones or salt which act as seals. Oil and gas accumulate only where the seal and reservoir rocks are in the right shapes and relative positions to form traps, which is called as the caprock . The migrating hydrocarbons fill the highest part of the reservoir, any excess oil and gas escaping at the spill point. Gas may bubble out of the oil and form a gas cap above it; at greater depths and pressure gas remains dissolved in the oil. Since few seals are perfect, oil and gas escape from most traps. In many fields incoming oil and gas balance this loss. Gas migrates and escapes from traps more readily than oil, but salt layers have proved to be a very efficient seal because salt contains no pore spaces and fractures reseal themselves.
The diagram below shows the main types of traps. Structural traps are formed by Earth movements which fold rocks into suitable shapes or juxtapose reservoir and sealing rocks along faults. Traps may also form when rocks are domed over rising salt masses. Stratigraphic traps originate where a suitable combination of rock types is deposited in a particular environment; for example, where a reservoir rock of permeable river sand is sealed by clays which accumulated in the surrounding swamps. In reality, most traps are formed by a more complex sequence of events and cannot be classified so rigidly. For example, in the reservoir rock was first folded and eroded, then sealed by an impermeable rock which was deposited later over the eroded structure. Where a particular set of circumstances has combined to produce a group of oil-or gas fields with similar trap structures or reservoir rock is termed a play. In order to trap migrating oil and gas, structures must exist before hydrocarbon generation ceases. All hydrocarbon fields are formed by a chance combination of events that produces the right sort of rocks and structures, together with the right timing.
A Oil in anticline | С Oil trapped by fault |
В Oil in rocks pushed up by a salt dome | D Oil in tilted rocks trapped by unconformity |
TERMS
Reservoir – porous rock (pores in rock are the spaces that occur between the individual rock particles; these spaces are created because the rock particles are irregularly shaped and so don’t fit together or exactly closely together.)
Porous – liquids and gas can be held and stored.
Permeable – means that liquid can flow through. A permeable rock has pores that are connected and so allows oil and gas to flow through.
Seal – oil and gas deposits remain in the reservoir rock if another layer forms on top is impermeable, i.e. does not allow liquids or gases to pass through or escape.
Trap – oil-containing rocks deep below the land or seabed in rock structures.
Fault trap – created when rocks slide past each other- an impermeable rock then forms a sort of dam trapping the flow of moving oil (a break or fracture in the ayers of rock in the earth’s surface).
Anticline - a place in the earth’s surface where layers of rock have formed an arch or dome.
Stratigraphic trap - a place in the earth’s surface where layers of one kind of rock come together, trapping a different kind of rock between them.
Salt domes – are formed by a combination of rock movements forcing a layer of rock salt up through the layers of rock above.
Basic types of traps
At first, the oil and gas only exist between the shale particles as extremely tiny blobs. Then, the intense pressure of the earth squeezes the oil and gas out of the shale, and the oil and gas fluids move sideways many, many miles. On their way, they may meet, up with other traveling oil fluids.
Finally, the oil and gas may become "trapped" in a rock formation like sandstone or limestone....a trap they can't escape! The oil and gas stay there, under tremendous pressure. After they are formed, oil and gas must be "trapped” in order to remain in place until it can be found. All oil and gas deposits are held in some sort of trap.
There are two basic types of traps:
Structural traps hold oil and gas because the earth has been bent and deformed in some way. The trap may be a simple dome, just a "crease' in the rocks, or it may be a more complex fault trap.
Stratigraphic traps are depositional in nature. This means they are formed in place, usually by sandstone ending up enclosed in shale. The shale keeps the oil and gas from escaping the trap. The stratigraphic trap can be formed when rock layers at the bottom were titled, then eroded flat. Then more layers were formed horizontally on top of the tilted ones. The oil moved up through the tilted porous rock and was trapped underneath the horizontal, nonporous rocks.
Petroleum Traps
A trap is any combination of physical factors that promotes accumulation and retention of petroleum in one location. Petroleum traps can be structural, stratigraphic or a combination of the two.
Structural traps
Deformation of rocks creates structural traps by folding or faulting. Many styles of traps result from deformation. Some styles are very complex because complicated stresses are involved in their formation. However, other styles are relatively simple because their patterns reflect uncomplicated stress regimes.
Anticline is a simple fold that traps petroleum in its crest. Anticlines form in a variety of ways, ranging from compression to gravity- induced fault block activity. As structural traps, anticlines are some of the most common productive structures. Producing anticlines can be symmetrical or asymmetrical structures. Many producing anticlines are faulted according to the stress patterns that formed them. Most anticlines are associated with some type of faulting in their deformational histories, whether that are normal or thrust-fault related. Faulted anticlines are usually asymmetrical. Overthrust anticlines produce abundantly from fields in overthrust belt provinces in the United States and Canada. In some cases, producing beds in overthrust anticlines are thrust against source beds of different ages not normally associated with the reservoirs. Overthrust anticlines are normally asymmetrical and complex faulted.
Faulting and tilting of crustal blocks produces a variety of trapping mechanisms. A single fault can provide a seal to prevent petroleum migration. Multiply faulted blocks create traps which are often connected, but can conversely, be entirely independent of each other. Petroleum production usually comes from the fault-sealed uothrown block. Enough petroleum comes from downthrown blocks to consider them important exploration objectives.
Fault traps involve up-dip closure of a reservoir bed against a sealing fault. A fault trap occurs when the formations on either side of the fault move. The formations then come to rest in such a way that when petroleum migrates into one of the formations, it becomes trapped there. Often, an impermeable formation on one side of the fault moves opposite a porous and permeable formation on the other side. The petroleum migrates into the porous and permeable formation. Once there, it cannot get out because the impervious layer at the fault line traps it.
Salt-related traps Salt structure formation can produce several different types of traps described by varying deformational intensities. Most salt structures are faulted in response to the degree of deformation. Traps related to salt deformation do not usually produce from the salt itself. They produce from beds deformed by the salt tectonics instead. Low-relief peripheral salt ridges form by alt migration down-dip from the up-dip limit of a salt wedge. Faulting in the overlying sediments occurs as a slight rollover produces a productive anticline in the beds overlying the salt. Salt flowage from a relatively thin marginal salt source into a salt pillow produces a low-amplitude productive anticline, or low-relief salt pillow in the overlying sediments. Faulting can be an accompanying deformational feature. Migration of salt from a somewhat thicker section into a salt pillow produces an intermediate – amplitude anticline in the overlying sediments. Faulting can accompany deformation of the upper beds. The final step in high-amplitude salt anticline deformation involves a thicker salt section and considerable faulting of overlying beds. Separation of overlying beds can occur as well. Faulting and folding related to upward and lateral salt movement produce a variety of traps. Salt overhangs and reservoir beds impinging against impermeable salt form other types of traps. Most salt movement accompanies sedimentation. This permits development of multiple source and reservoir beds throughout the depositional history of the sediments and the deformational history of the salt structure.
Stratigraphic traps when a trap is formed by depositional and sedimentary factors, it is considered a stratigraphic trap. The porosity, permeability and geometry of the depositional feature control the dimensions of the trap. Deformation is not a primary consideration in stratigraphic traps which exist because of their intrinsic depositional and geometric characteristics. Sand body traps: inasmuch, as there are numerous types of finite sand bodies, many different trap types are possible. Some trap types are modifications of each other because of changes in depositional environments. However, there are several well-defined types of sand bodies that form traps in many places (channel sands, delta reservoir traps, beach \ barrier bar traps). Reef traps are productive structures in many localities. Porosity in reefs provides highly prospective reservoir characteristics. A reef enclosed in a shale source that provides a hydrocarbon seal is a highly desirable exploration target.
Changes in porosity and permeability can be considered as a variety of depositional and diagenetic factors. Sandstones lose their permeability as it grades laterally. Hard, finely crystalline limestone becomes significantly more permeable when dolomized. A trap often develops where sand loses permeability or pinches out entirely.
Combination traps a depositional sedimentary body that has become formed forms a combination stratigraphic – structure if both stratigraphy and structure contribute to trapping mechanism. Since there can be many combinations of structure and stratigraphy, many different combined traps are possible.
Unconformity traps involve the proper combination of source, reservoir and sealing bed. Angularity in an uncomformable situation occurs when a sequence of horizontal beds is tilted, eroded and subsequently overlain by younger flat-lying beds. Tiltedpermeable beds surrounded by conformable and tilted impermeable beds and angularly overlain by flat-lying impermeable beds have potential as reservoirs. Hydrocarbons in the reservoirs move up-dip until they can move no farther against the flat-lying impermeable beds above the uncomformable surface. Deformation is responsible for the tilting of the reservoir beds in an angular unconformity. However, without the overlying sealing beds, there would be no trap.
A discomformity is an uncomformity which has no angular relationships between the flat-lying beds below and above the erosional surface. The surface of the uncomformity can be irregular. Trapping is accomplished by differences in permeability with sealing beds above the erosional surface, which may represent paleotopography. Sediments overlying a crystalline igneous or metamorphic terrain create a noncomformity. Sometimes the crystalline terrain is sufficiently weathered or fractured to provide permeability for hydrocarbon accumulation against the overlying sediments.
SUMMARY
Дата добавления: 2019-01-14; просмотров: 474; Мы поможем в написании вашей работы! |
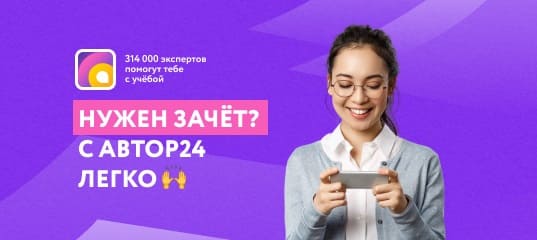
Мы поможем в написании ваших работ!