Topsoil of Stagnic Cambisols (Dystric)
Changes in Physical and Chemical Soil Characteristics as a Result of Subsurface Tile Drainage
Introduction
Agricultural subsurface drainage, popularly known as tile drainage, is an essential water management practice in agricultural regions with seasonal, high water tables 1 . It is commonly used to improve the poor drainage capacities of agricultural fields 2 . In some areas of the temperate zone, drainage systems continue to be an essential tool of groundwater control 3 . This practice reduces the risk of water logging and soil erosion caused by surface runoff 2 and it ensures the most favourable development of plants and suitable conditions for tillage. Drainage stabilizes productivity by minimizing the variation in crop yields from year to year, and it results in a considerable reduction in the duration of over-wetting and over-flooding of the active soil layer 4 . The greatest drainage importance in the control of the soil water regime is in the winter and spring periods 5 . To achieve all these aims, drainage systems should be properly designed and adequately installed using appropriate materials 3 .
Subsurface drainage has been practiced for thousands of years; however, rapid introduction in Europe and North America only started around 1940, when the prevailing empirical knowledge of drainage and salinity control gained a solid theoretical foundation. During the second half of the 20th century, drainage was implemented in about 150 million ha of under-producing and naturally waterlogged or saline lands. This resulted in important improvements that contributed to a considerable increase in food production. Drainage has also contributed to agricultural intensification and diversification and as such has made the agricultural sector more competitive and financially sustainable 6 . In the Czech Republic, 25% of the soil agriculture fund is drained; that is, an area of 1,084,000 ha of soil 7 .
The drainage of agricultural soils was intended to improve the water and air conditions of soils. Therefore, it is very important to describe the changes in the physical and chemical properties of the soil affected by this ameliorative construction.
Materials and Methods
The Zelezna model area is located in the Domazlice district on the border with Germany (49˚34 - 35'N, 12˚34 - 36'E). From a geological point of view, it belongs to the crystalline or moldanubic massif of the Bohemian Forest (Cesky Les), with a predominance of cordirietic gneiss and silmanitic-biotitic migmatised paragneiss with cordierite. The area falls within the moderately cool and wet climatic region 8 .
|
|
The majority of its soil cover (approx. 40%) consists of Stagnic Cambisols (Dystric), Haplic Stagnosols make up about a quarter of the soil cover, Haplic Gleysols almost one fifth, and Endogleyic Stagnosols one tenth. The remainder of the soil cover consists of Histic Gleysols, Cambisols (Dystric), and GleyicStagnosol 9 . The drainage network comprises natural streams (the Nivni, Farsky, and Lesni), and man-made canals. The study area lies within the drainage basin of the Danube. In the 1970s, the area was drained systematically using subsurface tile drainage with the aim of improving the physical state of the soil and water regime of the agricultural land.
A detailed hydropedological survey was carried out in the 1970s by the State Land Reclamation Authority 1011 to provide a background for the drainage construction. The previous data of soil properties before drainage were found in The Comprehensive Soil Survey of Czechoslovak Soils and other soil investigations in the model area 121314 .
The systematic pipe drainage system was formed by flexible plastic perforated pipes (we obtained the original construction plans). Due to a susceptibility to silting, the pipes were covered with glassfabric. The spacing of the drains depended on the water-logging level of the soil and varies between 7.5 and 11 m, and the depth of the pipe placement was 1m. The system is still functional. After the drainage had been built, the agricultural areas were used as arable land, but since the 1990s they have been used as pasture (Figure 1). The fertilization of the Zelezna study area is extensive analogous to farming intensity. Carbamide is applied during spring time on the pastures (trefoil grasslands). The grasslands are fertilized by potassium with a fertilizer rate of 50 kg/ha and by phosphorous with a rate of 30kg/ha during their reclamation.
The detailed hydropedological survey was carried out in Zelezna before drainage construction in 1974 (old data). The new soil samples were taken in 2006 at the same places as the former soil pits in 1974 (new data). These two sets of data were subsequently statistically compared (T-test). We determined the same physical characteristics as in 1974 and used the same analytical procedures as in the first datasets to have two comparable sets of data. The results were processed by the MS Access database program and statistically evaluated by a paired t-test at a significance level of α0.05 or α0.1 (in this case, the index is attributed in the table) in the Unistat program. We performed this process for the topsoil and also for the subsoil for two of the most widespread soil types-Stagnic Cambisols (Dystric)-11 soil pits, and Haplic Stagnosols-6 soil pits.
|
|
From each soil pit we took a disturbed sample to evaluate the soil chemical properties, namely Exchangeable soil reaction pHKCl 15 the Percentage of soil organic matter SOM 1617 the Cation exchange capacity CECpH8.1 18 the Effective cation exchange capacity ECEC 19 and the Saturation of sorption complex (calculated value).
In addition, we took away 3 undisturbed soil samples from each horizon, we set aside samples that were statistically different, and then we calculated the average value from the rest of the samples. The undisturbed samples were taken by steel cylinders of 100 cm3 (Kopecky’s rings) to determine the following soil physical properties: Particle density (PD)―the weight of a unit volume of soil without pores 20 , Bulk density (BD)―the weight of a unit volume of dry soil 21 and Maximum capillary water capacity (θCMC)―% volume of capillary soil pores which corresponds approximately to the field water capacity (θFC), determined in the laboratory using an empirical procedure according to Novak 22 . Its determination is based on the mass change after 2 h water suction from Kopecky’s ring fully soaked with capillary water on dry filter paper. The last physical property is Porosity (P) which is defined as the volume of the sample in natural deposition not filled with solid particles, established by means of the formula:
P=(PD−BD)/PD*100P=(PD−BD)/PD*100
The basic soil characteristics of the compared soils are shown in Table 1.
|
|
Results and Discussion
The statistical evaluations of data sets are shown in Table 2 and Table 3.
Table 1. Basic average soil characteristics of the Zelezna model area at present.
Table 2. Changes of soil properties of Stagnic Cambisols (Dystric) (n = 11).
Explanations: Ø―statistically inconclusive; ▲―statistically conclusive increase; ▼―statistically conclusive decrease.
Table 3. Changes of soil properties of Haplic Stagnosol (n = 6).
Explanations: Ø―statistically inconclusive; ▲―statistically conclusive increase; ▼―statistically conclusive decrease.
Topsoil of Stagnic Cambisols (Dystric)
There was a statistically conclusive increase in the pH value in the topsoil of Cambisols during the last 30 years. Despite this increase, all soil pits in the model area were still acidic or strongly acidic. The increase in the value of soil reaction was probably caused by ameliorative liming, which was applied after the drainage construction, and by liming of the area during intensive farming. It was also caused by the decreasing of soil acidification, because there is only better humification of the soil organic matter in sufficiently aerated soils. Thus, it prevents production of fulvo acids which decrease the soil pH value.
The soil organic matter (SOM) content statistically increased in the topsoil of Cambisols. During intensive cultivation of the arable land there was a higher loss of soil organic matter compared to the areas extensively managed. The area of previous soil pits have been used as pasture during the last 15 years, so vegetation consists of grasses or a grass-legume mixture. The increase of the SOM was probably caused by the accumulation of organic matter in the topsoil (remediation of vegetation, better humification...) after the land use change. It is very interesting that there was a more significant increase of the SOM in almost all the soil pits taken during the hydropedologic survey in 1974 on the arable land compared to the other soil pits. This was caused by the already lower SOM content in these soils before drainage construction, due to losses of soil organic matter during their more intensive land use.
|
|
The value of the cation exchange capacity (CEC) characterises soil sorption ability. There was a statistically conclusive decrease in this characteristic in the topsoil of Cambisols. The change of content of the effective cation exchange capacity (ECEC) was statistically inconclusive over 30 years. We can state that, except for 2 soil pits, there was a decrease in the value of the effective cation exchange capacity, but the change was not conclusive.
The saturation of sorption complex is defined as the ratio of the value of the effective cation exchange capacity to the cation exchange capacity. There was a statistically significant increase in the value of this characteristic. According to the saturation value, most soils belonged to the same evaluated group as in 1974. The soil pits were mostly taken on the upper parts of steep terrains. The decrease in the value of the CEC could be caused by decreasing one of the content of sorption complex during intensive farming. Whereas the soil organic matter content of most of the soil pits increased, there must have been a decrease in the content of fine particles, probably washed off by erosion. Simultaneously, there should have been a decrease in the content of organic matter, which was not shown in our results. So the intensive erosion must have occurred in the period after drainage. In the nineties, the arable land was converted to permanent grassland. After stabilisation of the soil by grassing, the erosion features and accumulation of organic matter were eliminated.
Concerning the physical soil characteristics of the topsoil of Cambisols, there was only one statistically conclusive change-the increase of minimum air capacity. The increase of this value was caused by drainage, and thus it enabled air to enter the soil pores. Apart from this change, some trends and continuities were found in the physical characteristics. Four soil pits showed an increase in porosity after drainage, which was probably caused by better biological activities and the higher presence of plant roots in the topsoil. The soil structure was stabilised by the activities of soil flora and fauna, which was confirmed by an increase in the values of minimum air capacity. Along with these changes, there was also a decrease in the maximum capillary capacity. With regard to the increase in porosity, there was probably an increase in the number of macro pores. The soil pits which showed an increase in porosity value also indicated an increase in bulk density. The decrease in porosity compared to the soil samples taken in 1974 was shown in the soil pits which were significantly influenced by water. There were significant marks of pseudogleyzation by means of the frequent appearance of Fe-Mn nodules in the topsoil. The soil structure of waterlogged soils was less stable according to our conclusions and after drainage caused the disintegration of structural elements, which consequently had an influence on the decrease in porosity. This was confirmed by the increase in particle density and bulk density.
Дата добавления: 2018-02-28; просмотров: 294; Мы поможем в написании вашей работы! |
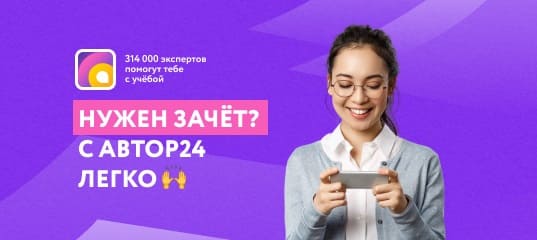
Мы поможем в написании ваших работ!