Some physical properties of C subgroup elements
Summary of general characteristics
Si is the closest analogue of carbon in the group. Silicon compounds are the mineral basis of Earth and play a role, similar to the role of carbon compounds in the development of life.
Ge, Sn, and Pb are more similar in their chemical behavior than typical elements (C and Si). In the series Ge—Sn—Pb the number of energy levels, Ra increase and their metallic nature grows, respectively: Ge is a non-metal, Sn and, especially, Pb have metallic properties.
Electron configurations of carbon subgroup
IV group p-elements include C, Si, Ge, Sn, Pb.
General formula of outermost shell configuration of these elements in the ground state is ns2np2. Having two unpaired p-electrons, they can display the valence II (this trend is strengthened towards Pb). However, the excitation of the 4 unpaired valence electrons takes place easily. The valence IV occurs in this case. It becomes the most typical state of C and Si and its stability diminishes toward Pb.
The carbon subgroup includes two elements (C, Si), which are, respectively, the core of living organisms and inanimate nature.
As the size of the atom increases the ionisation energy and other physical constants of the elements alter (see Table below) and these changes are reflected in the gradual change from a typical nonmetallic element, carbon, to the weakly metallic element, lead.
Elemental C and Si are typical non-metals that have the atomic lattice with covalent bond. They have elevated hardness, melting and boiling points.
The values of these constants are reduced significantly in case of Ge, but they are still relatively large. Together with the fragility, it characterizes Ge as a diamond-like crystal with covalent bonding mechanism. At the same time, Ge has some contribution of metallic bonding. Its forbidden band energy gap essentially diminishes and conductivity elevates.
Semiconductor properties of Sn exist only below 13,2 °C (a -Sn, D E = 0.08 eV), at higher temperatures it has transition into the metallic ß-Sn allotrope. Pb is a metal, which does not display semi-conductive properties.
Growth of metallic properties is accompanied by a consecutive reduction in elements’ ionization energy, electronegativity.
Silicon. The valence electrons configuration of silicon is 3s23p2 (3d0). It differs from C atom due to 3d- vacant sublevel that leads to significant differences in behavior of these elements.
Compare bond energies of silicon with other elements:
|
|
Element | Bond energy , к J / mol | |||||||||
C | Si | H | O | F | Cl | Br | I | N | S | |
C | 348 | 328 | 414 | 359 | 488 | 340 | 286 | 214 | 207 | 272 |
Si | 328 | 177 | 320 | 445 | 568 | 382 | 310 | 236 | 331 | 264 |
Bond energy of valence electrons with Si nucleus is considerably smaller than in C due to larger RA. Therefore, Si—Si bond is much weaker comparing with C—C (177 and 348 kJ / mol). Silicon p-orbitals do not form p-bonds, and multiple bonds Si = Si or Si º Si, respectively. For these reasons, to break Si atoms chains is easier than carbon ones.
However, additional p -bonding is realised in Si at the expense of the overlapping of its own free d-orbitals with twoelectronic orbitals of partners, as a result their energy is higher. Another reason for this growth in energy is attributed to the growth of ionic bonding contribution (EN difference of interacting elements is increased).
Thus, unlike carbon, Si has no so strong bonds with hydrogen. On the contrary, its bonds with O are much stronger. Therefore, there is a sharp decrease of the number of stable Si compounds with hydrogen compared to hydrocarbons and, simultaneously, diversity of Si compounds with O. These distinguishing features of silicon influence upon comparative variety of organic and mineral world: more than a million species of living organisms, on the one hand, and about three thousand different minerals, on the other hand.
Selected properties of the elements and their simple substances are shown in the Table below.
Germanium, tin, and lead. Electronic configuration of atoms in the ground state is ns2np2, like typical group elements (C and Si). On the other hand, Ge and Sn have these sublevels after completed (n-1)d10-sublevels, Pb has it after 4f145d10-sublevels. This circumstance greatly affects the properties of elements. Since 3d-and 4f-sublevels are caino symmetric, there is a significant contraction of atoms in elements where they are formed.
Caino symmetric (Greek “kaynos” — new, that is a new type of symmetry of orbitals) are called orbitals, which appear for the first time. They include 1s, 2p, 3d, 4f-orbitals etc. The radial distribution of electronic density for them contains single characteristic peak (without many peaks of the second order of magnitude!).
|
|
Unlike cainosymmetric, the other orbitals of the same symmetry have additional maxima on the electronic density radial distribution dependence. This leads to larger attraction of cainosymmetric electrons to the nucleus due to a significant weakening of screening effect, reducing orbital atomic radii, increase of ionization energy and, consequently, weakening metallic properties cainosymmetric elements compared with the other elements.
Contraction effects make difficult transition into the excited state:
ns2np2 ® ns1np3
and reduces ns-electrons capability of forming chemical bonds. Therefore, in the series Ge—Sn—Pb decreases the highest oxidation state (+4) stability and increases stability of oxidation state (+2). Oxidation state +4 of germanium is significantly more characteristic; both oxidation states of Sn: +2 and +4 are approximately equal in their stability.
Some physical properties of C subgroup elements
C (diamond ) | Si | Ge | b -Sn | Pb | |
Atomic radius , nm | 0,077 | 0,118 | 0,139 | 0,158 | 0,175 |
m . p ., ° С | 3500 | 1415 | 958,3 | 231,9 | 237,4 |
b.p. , ° С | - | 3250 | 2850 | 2620 | 1745 |
hardness , kg∙mm-2 | 104 | 980 | 385 | 30,2 | 3,9 |
density , g∙ с m-3 | 3,51 | 2,33 | 5,35 | 7,29 | 11,34 |
S298 ° , J∙mol -1 ∙K -1 | 2,37 | 18,8 | 31 | 51,48 | 64,81 |
Forbidden band gap , е V | 5,2 | 1,08 | 0,78 | - | - |
Electroconductivity ( Hg=1) | - | - | 0,001 | 8 | 5 |
Ionisation energy , е V | 11,26 | 8,151 | 7,899 | 7,344 | 7,417 |
Electronegativity | 2,5 | 1,8 | 2,02 | 1,72 | 1,55 |
Е ° М2+/М 0 , V | - | - | 0,00 | -0,136 | -0,126 |
Physical properties
Carbon
|
|
1 2 Comparison of crystal lattice of atomic structure in diamond (1) and layered structure in graphite (2) | Some allotropes of carbon: a) diamond; b) graphite; c) lonsdaleite; d–f) fullerenes (C60, C540, C70); g) amorphous carbon; h) carbon nanotube |
T here are four allotropic modifications: diamond, graphite, carbin and fullerene (C60).
Diamond. In nature, it is formed at large depths at enormous pressures and high temperatures. In some places on Earth it “walked” up a surface in so called cimberlite tubes (from the name of small town Cimberli in South Africa).
The content of diamonds in rocks is very small. So, in the richest Cimberly mine Capa from 2 ton of rocks are obtained 1 g of diamonds. Mass of diamonds is expressed in carats (1 carat = 0,205 g). The greatest from all other diamond named Coullinan (3024 carat) was found in South Africa. Clear transparent diamonds are met rarely (~ 5%). Diamond crystals are processed, forming it into definite facets, and then they are jewels - diamonds. Cloudy, leaden-grey diamonds are named bort. A greater part of all diamonds obtained consists of it.
A diamond has an atomic face-centered cubic crystal lattice in which every atom of carbon has tetrahedral configuration of surrounding atoms. Crystal of diamond can be considered as an enormous polymeric molecule C¥, in which every atom of carbon is found in the sp3-hybridization state and is connected with the neighbour atoms by strong two-electronic covalent bonds.
Distance of C—C = 0,154 nm. Density - 3,51 g/cm3. Diamond is absolute dielectric.
Graphite. It is soft black material, easily scratched by a nail, it is “fat” at touch with a finger. Its density is considerably lower, than of diamond = 2.1-2.3 g/cm3.. It is good conductor of heat and electricity. Graphite is most thermodynamically stable modification of carbon (heat of its combustion is on 0.84 kJ/mol lower than the heat of combustion of diamond).
For graphite is characteristic hexagonal crystalline structure. It has a plane-stratified structure:
|
In the plane of the lattice every atom of carbon is bound to the three neighbours by covalent bonds. The length of bond is less (and also stronger), than in the diamond = 0,142 nm, bond energy is 716 kJ/mol. Distance between parallel grid planes is comparatively large - 0,3345 nm. Bonds between planes are weak; the order of magnitude of intermolecular forces is about 17 kJ/mol. For this reason the hardness of graphite is low (easily slide along the planes).
|
|
An atom of carbon in graphite is found in the sp2-hybridized state, and the monoelectronic pz-orbital remains atomic. Like in C6H6 such orbitals are involved in formation of multicentral delocalized p -bond. However, if in C6H6 this bond is six-centered, in graphite it is delocalized within bounds of enormous number of atoms of carbon of one crystal lattice. Such bond reminds metallic, therefore graphite has large conductivity in the direction of planes. Interestingly, that athwart to the planes of cristallic lattices graphite is a dielectric. It is a good example of anisotropy of crystals.
Carbine. Prepared by V.V. Corshac at catalytic C2H2 oxidation in 1963. It is a linear polymer.
a -carbine –CºC–CºC–Cº (poliine) and
b -carbine: =С=С=С= (polycumulene) exist.
In this compound sp-hybridization of atoms of carbon is realised. Carbine is black fine-crystalline powder (density 3,23—3,3 g/cm3) with semiconductor properties. Distances between chains in carbine (0,295 nm) are less than between graphite layers.
Differences: a-carbine is oxidized by ozone to oxalic acid; b-carbine to coal. Carbine is found in nature as mineral chaoite.
Fullerene. At the beginning C60 molecule was discovered in 1990 as a constituent of new carbon allotrope, which was named fullerene.
It is produced at evaporation of graphite by voltaic arc in helium atmosphere. Two forms were isolated: yellow-brown fullerene, C60, and red-brownish cluster C70. It has uncharacteristic for allotropes of carbon property to be dissolved in various organic solvents (benzene, hexane etc.). Its density (1.65 g/cm3) is only the half of diamond density, m.p. 360°C. Fullerene is absolutely stable on air.
Fullerene has a shape of soccer ball that consists of 20 hexagons and 12 pentagons. Two hexagons and one pentagon are together at one top. The length of bond C—C in a rib, which is common for two neighbouring cycles, makes up 0,1432 nm, and between five- and six-membered cycle - 0,1388 nm. Every atom of carbon has sp2-hybridized state, and one-electron pz-orbital, as well as in graphite, forms nonlocalized p-bond that includes all cluster molecule.
The radius of its intramolecular cavity exceeds 0,500 nm. This is enough for placing inside any atom and even small molecules. Such compounds form at evaporation of graphite. Therefore, scientists were disturbed that they could be formed during Chernobyl disaster, where graphite reactor was burned. Long-life radioactive nuclides that have penetrated inside the fullerene sphere could cause the catastrophic consequences because carbon cluster easily passes through biological membranes of biological cells. The modern researches of fullerenes properties are very intensive.
Silicon. Elementary Si is a transparent crystalline substance with face-centred cubic diamond-like lattice. The lattice has the following parameters:
density 2.328 g/cm3,
m.p. 1415 ° C
b.p. 3250 ° C
hardness (The Moos scale) - 7.
Si orbitals in its crystal are in the state of sp3-hybridization. Compared with carbon, silicon has larger atomic radius, so the length of Si—Si bonds in crystals is larger than C—C, and the strength is lower. Therefore, crystalline Si has lower hardness and melting point than diamond.
Si a typical semiconductor with energy gap 1.08 eV. Its valence band is formed by 3p-orbitals, the conduction band — by 3d-orbitals. With temperature growth, some electrons can shift to the conduction band and create an electrical current. Its carriers in the conduction band are electrons in the valence band carriers of charge are holes.
There is also a powdered amorphous Si. Investigations of recent decades have shown that it actually consists of small crystallites of a cubic silicon and some impurities.
Si is one of the least electronegative elements among non-metals. Its EN is 1.8 and this parameter is close to electronegativity certain metals.
Natural Si consists of 3 isotopes: 28Si (92.27%), 29Si (4.68%), 30Si (3.05%).
Germanium, tin, and lead. By its physical properties, Ge is similar to silicon; Sn and Pb are typical metals of white and blue-gray colour. Hardness and brittleness drops fast in the series Ge—Sn—Pb: Ge is extremely hard and brittle, and Pb can be scratched and rolled into thin sheets. Sn occupies an intermediate position. Sn and Pb are low melting elements:
m.p. (Sn) = 231.9 oC; m.p. (Pb) = 237.4 oC, while Ge m.p. = 937.1 oC.
![]() |
Allotropes. Ge has similar to diamond allotropic form. It is greyish-white and brittle substance. Crystalline Ge is a semiconductor (n-type) with energy gap width, D E = 0.785 eV.
Pb has only one metallic modification. Sn forms three allotropes. b -Sn differs significantly by its density; it has a close-packed structure of metals, density of which is significantly higher than that in the structure of diamond. At transition to g-Sn density drops, it becomes fragile, and easily grinds into the powder.
Transition a -Sn Û b -Sn proceeds with very low rate. Transition b -Sn Þ a -Sn accelerates lowering temperature and the rate becomes maximal at -33 °C. Especially fast is this transition at the presence of a-Sn, which particles are nuclei of crystallisation. This transformation is called “tin plague”. Since the specific volume of a-Sn is larger by 25.6% materials containing metallic Sn are transformed into powder at low temperature.
History Of Discovery
Carbon was known to the humanity from time immemorial in the form of charcoal which was used for heating, extraction of metals from ores, for medical treatment. It has got the modern name “carboneoum” (from Latine carbo “a coal”) and authentication as a chemical element in 1787.
Silicon. Silicon was first identified by Antoine Lavoisier in 1787 (as a component of the Latin silex, silicis for flint, flints). The first elemental Si samples were extracted in 1811 by Gay-Lussac and Thénard. They passed vapours of SiF4 over heated potassium. However, they didn’t know certainly what was the product formed. Properties of Si were described by J. Berzelius in 1822. He called the new element Si (lat. Silex - Si) and gave him the symbol Si.
Germanium. German scientist C. Winkler discovered Ge in 1886 conducting chemical analysis of the new mineral arhyrodite, 4Ag2S•GeS2. The existence of this element was predicted D. Mendeleev in 1871. He called this element ekasilicon and predicted its properties with high accuracy. D. Mendeleev named C. Winkler the strengthener of the Periodic law.
Clemens Winkler Dmitry Mendeleev
Tin and lead. Metallic Sn and Pb have been known since ancient times. Their articles were found in ancient Egyptian tombs. Generally, Sn alloy with Cu (bronze) played a huge role in history of our culture. Pb had been used in ancient Rome in large amounts to produce water pipes, lead white, minium and other useful substances.
OCCURRENCE
Carbon. The natural carbon consists of two isotopes 12C (98,892%) and 13C (1,108%).
According to the International convention of 1960 12C isotope is chosen as a standard for computation of relative atomic masses.
Composition of natural carbon includes in trace quantities also a slightly radioactive isotope 14C, which is continuously formed in mid air from atoms of N under bombardment with cosmic rays.
Calculations show that about 9,8 kg of 14C forms annually in the atmosphere. Its period of half-decay is 5300 years. As for the last 20000 years the intensity of space radiation remains unchanging, formation of radio-active carbon is constant. In air it reacts with O2, transforming into CO2, and at photosynthesis it is consumed by the plants. Through them with a meal it enters in the organisms of living creatures. At dying of plants and living organisms off the isotope not anymore accumulated, but begins to decompose gradually. According the rest content of 14C in the relics of organisms died long ago it is possible to define precisely enough the period of their life. This technique has found application in archaeology, paleontology, oceanography and other fields of knowledge. The term of life is determined then according the formula:
t = 8040 ln (14/ A), where: A – nuclei 14C decay number per minute; t - age, in years.
There are a lot of forms of finding of carbon in nature, it is contained in:
· tissues of living organisms and products of their destruction (anthracite coal, petroleum, peat);
· minerals, mainly carbonates. Most widespread mineral is calcite - CaCO3 (it forms enormous deposits known as limestone, marble, chalk and etc.). Also magnesite MgCO3, dolomite CaCO3•MgCO3 and heavy spars FeCO3, MnCO3, ZnCO3. More rarely are met in nature malachite Cu2CO3(OH)2 and soda Na2CO3. Natural carbonate rock depositscontain in itself a greater part of the Earth natural carbon (more than 99%).
· a significant quantity of carbon in a form of CO2 is found in the atmosphere (~ 0,03 vol.% = 2000 billion tons).
· in a very small quantities the free carbon is met in the forms of graphite and diamond.
Silicon content in the Earth crust makes up 27.6% by mass. It is found only in compounds. The most common compound of silicon in nature is silicon dioxide, SiO2 (silica). This composition have ordinary river sand, quartz, sometimes occurring semiprecious morion, citrine, amethyst, agate, jasper, which are colourful oxides of different metal.
Significantly larger fraction of SiO2 (about 43% by weight of the Earth's crust) is chemically bound as silicates and aluminosilicates (kaolin, clay and a variety of rocks, usually granite). The latter is a natural mixture of quartz, mica and feldspar. As a whole, more than half of the crust consists of SiO2.
Germanium, tin, and lead are not common elements, their content in the Earth's crust: Ge - 7∙10-4, Sn - 8∙10-3, Pb - 1,6∙10-3 % wt.
Ge is a rare element found in trace quantities in coke obtained from bituminous coal. The predominating part of germanium is extracted from polymetallic copper-zinc and zinc ores. It has no own minerals that are commercially valuable.
Minerals that are rich in Ge:
germanite Cu2S∙CuS∙GeS2
arhyrodite 4Ag2S∙GeS2
occur very rarely.
The most important minerals of Sn and Pb are cassiterite (tinstone), SnO2, and galena, PbS
USES
Carbon. Diamonds. They are applied for technical purposes - boring drilling, polishing of especially hard materials, cutting of glass, making of bearing, for precise instruments. For all this purposes the diamond is suitable thanks to its extraordinary hardness. Diamonds exceed all other materials on hardness.
Tungsten carbide milling bits
Silicon. As the second most abundant element in the earth's crust, silicon is vital to the construction industry as a principal constituent of natural stone, glass, concrete and cement. Silicon's greatest impact on the modern world's economy and lifestyle has resulted from silicon wafers used as substrates in the manufacture of discrete electronic devices such as power transistors, and in the development of integrated circuits such as computer chips. It has photovoltaic applications.
Production
Carbon. Amorphous carbon, diamond, and graphite are produced for industrial purposes.
Diamond. In our time in considerable volumes diamonds are obtained artificially, in particular in Kiev. Artificial diamonds are very small and not transparent. Therefore, they are used in industry. The process of their formation from graphite occurs at temperatures about 3000 ° , pressures about 100 thousand of atmospheres in the presence of catalysts and in definite reaction mediums.
Graphite: coal that contains quartz (SiO2) is heated in electric furnaces by electrical current of a few thousand amperes during 12-24 hours. At these conditions graphite crystals are grown from the molten mixture.
Amorphous coal (black carbon): wood or other organic matters are heated at high temperatures without access of air. Coke, wood char-coal, bone coal, animal, blood coal, soot are of great importance for industry types of “amorphous carbon”. Soot is prepared at incomplete combustion of organic substances:
CH4 + O2 = C + 2H2O
In laboratory: incineration of magnesium in the atmosphere of carbon dioxide:
CO2 + 2Mg = 2MgO + C
Silicon. Si is produced in industry mostly as an alloy with iron (ferrosilicon) by strong annealing the mixture SiO2, iron ore and coal. Content of Si is varied in the range 9-95%. Alloys with less than 20% of Si are produced in blast furnaces, and more than 20% Si in electric furnaces. It is used to remove dissolved oxygen from molten metal, as well as alloying components in the production of acid enamel.
In industry:
1. Technical Si (up to 99%) for silicothermy:
SiO2 + 2C Si + 2CO (in electric furnaces, coke)
2. Pure (semiconductive) Si.
SiCl4 + 2Zn Si + 2ZnCl2,
or
SiCl4 + 2Н2 = Si + НCl,
Crystals of semiconductive Si with required electrophysical and structural properties can be grown (the method of zone melting).
3. Especially pure silicon is produced by silane, SiH4, decomposition at 600-700°C:
SiН4 Si + 2Н2,
In a laboratory:
Dry sand with powdered magnesium mixture is ignited by Mg ribbon:
SiO2 + 2Mg = Si + 2MgO
After cooling, the mixture obtained is sequentially processed by HCl (to eliminate MgO) and HF (to dissolve excess SiO2).
Germanium. Firstly, GeCl4 is obtained that hydrolyzes further to GeO2. The latter is reduced by hydrogen:
GeO2 + 2H2 Ge + 2H2O
Annual production of Ge achieves several hundred tons. Semiconductor technologies are the major applications of Ge, satisfying the requirements for the extremely high purity (impurities should not exceed about 10-9 at. %).
Tin. Tin ore is initially concentrated by flotation and then reduced by coal (Al or Zn can be also used):
SnO2 + 2C = Sn + 2CO
Lead. In the extraction of lead, the sulfide ore is first roasted together with quartz in a current of air:
2PbS + 3O2 = 2PbO + 2SO2
¯6e 2e•2
Any lead(II) sulfate formed in this process is converted to lead(II) silicate by reaction with the quartz. The oxide produced is then mixed with limestone and coke and heated in a blast furnace. The following reactions occur:
PbO + C =Pb + CO
PbO + CO =Pb + CO2
PbSiO3 + CaO + CO =Pb + CaSiO3 + CO2
Crude lead contains traces of anumber of metals.
Tin and lead of high purity are produced by electrorefining of these metals (during electrolysis SnSO4 and Pb[SiF6] solutions by making the crude metal the anode in an electrolytic bath). A smooth coherent deposit of lead is obtained on the pure lead cathode when the current is passed. The impurities here (i.e. all other metals) form a sludge in the electrolytic bath and are not deposited on the cathode.
The annual production of Sn and Pb in the world achieves hundreds thousands tons.
CHEMICAL PROPERTIES
Carbon
In the ground state, electronic configuration of carbon is 1s22s22p2:
p p
s s
401 kJ
In this state it forms three covalent bonds, two of which are formed according exchange mechanism, and one on donor-acceptor (such bond is in CO). But carbon rarely is bivalent. Excitation of its atoms occurs easily and forms a state with 4 unpaired electrons. Energy required for atom excitation is exceeded by the energy released at formation of two additional covalent bonds. A difference between s- and p-electrons is taken off by sp- sp2- or sp3-hybridization, to which is undergone an atom of carbon depending on a partner in chemical bond. Therefore, mostly carbon behaves like fourvalent element. As d-orbitals in it are absent, its maximal valence is also 4.
Identical number of valence electrons and valence orbitals takes place only at two elements - hydrogen and carbon. For this reason they can form the greatest number of compounds between itself and with other elements.
![]() |
It must be taken in mind that s-bonds between atoms of carbon are most strong in comparison with the homoatomic bonds of other elements, which are able to form similar chains:
kJ·mol-1
It predetermines extraordinary abundance and variety of derivatives of carbon. From heterobonded atoms of carbon most widespread are C—H as a result of their large strength (441,2 kJ/mol). Carbon is also able to form multiple bonds.
With EN=2,5 carbon is an intermediate between electropositive and electronegative elements, although it is nearer to the last. Therefore even at the maximal polarization of atoms in its compounds there are no free ions C4+ and C4-. Effective charges on atoms of carbon in all known compounds are considerably less then 1, that is compounds of carbon are weak polar.
In the simple substances carbon has already used its valence electrons, creating polymeric structures of diamond or graphite. Therefore in its crystalline forms carbon is absolutely chemically inert.
From this, regardless of modification, carbon has neither a taste nor smell, it is extraordinarily heavily melted and evaporates, it does not dissolve in all ordinary solvents. It is well dissolved in many molten metals (Fe, Co, Ni, the Pt metals) only, and at cooling it is again crystallized in the form of graphite.
Its chemical activity rises at transition from the diamond to amorphous carbon, which can be ignited in the atmosphere of oxygen at comparative slight heating:
С + О2 = СО2 DН°298 = –395 kJ/mol
With a fluorine an amorphous carbon interacts already at ordinary conditions:
C + 2F2 = CF4
At high temperatures carbon reacts with metals, N2, O2, S, Si, B, with oxides of metals and salts, for example:
C + 2S = CS2
C + CuO = Cu + CO
CHEMICAL PROPERTIES
Silicon
Elemental Si, especially crystalline, is chemically inactive at STP. It is not soluble in acids. More active amorphous Si reacts with the mixture of HF and HNO3(conc):
3Si + 4HNO3 + 18HF = 3H2[SiF6] + 4NO + 8H2O
( HNO3 oxidizes Si to SiO2 , and SiO2 with HF form complex).
Si dissolves easily even in dilute solutions of strong alkalis:
Si + 2NaOH + H2O = Na2SiO3 + 2H2
Amorphous Si directly combines with F2, at t= 400-600 ° with O2, Cl2, Br2 and S, and at higher temperatures - with N2 and carbon.
Silicon has strong affinity for oxygen (DH ° = -908 kJ / mol) that is why silicothermy is used in the extraction of metals from their compounds. Silicon in these reactions plays a role of reducing agent:
2Cr2O3 + 3Si + 3CaO 4Cr + 3CaSiO3
2V2O5 + 5Si 4V + SiO2
CHEMICAL PROPERTIES
Germanium, tin, and lead
In the series Ge—Sn—Pb the growth of Ra is observed and metallic properties are strengthened. Ra(Ge) is only slightly larger than Ra(Si), which is above it, therefore, the chemical activity (reducing properties) of simple substances of Ge is low.
Oxidation state (+2) stability grows down the group:
Ge(cryst) + GeO2(cryst) = 2GeO(cryst) DGo = 83 kJ/mol
Sn + SnO2 = 2SnO DGo = 8 kJ/mol
Pb + PbO2 = 2PbO DGo = -159 kJ/mol
Ge+2 ion is a strong reducing agent, and Pb4+ is a very strong oxidising agent, so, for example, co-existence of ions I-1(reducing agent) and Pb4+ is impossible; in other words, compounds PbI4, and PbBr4 do not exist, and PbCl4 is extremely unstable.
The change of oxidation states stability from (+4) to (+2) in the series Ge—Sn—Pb can be seen in the reactions with oxidants:
Interaction with O2:
Ge + O2 GeO2 (strong heating)
Sn + O2 SnO2 (slight heating)
2Pb + O2 = 2PbO (metallic Pb is always covered with protective oxide film)
Interaction with chlorine:
Ge + 2Cl2 GeCl4
Sn + 2Cl2 = SnCl4 (at STP)
Pb + Cl2 PbCl2
Interaction with sulfur:
Ge + 2S GeS2
Sn + S SnS (but there is also SnS2)
Pb + S PbS (not PbS2, because Pb4+ is a strong oxidising agent).
Ge, Sn, and Pb reactions with acids are significantly different. Ge (is situated after H2 in the electromotive series of metals) does not replace H2 from acids. Sn and Pb (both are situated before H2 in the electromotive series) form soluble salts of divalent elements at the action of strong non-oxidant acids (HCl, diluted H2SO4 etc.):
Sn (Pb) + 2HCl = Sn(Pb)Cl2 + H2
The low oxidation state (+2, not +4) of metals takes place because H+ ion is not a strong oxidant (the reaction 2H+ +2e = H2, Eo = 0 V) and H2 formed is a reductant. The reaction of Pb with HCl is slow due to the formation of surface film of insoluble PbCl2. The reaction rate increases in concentrated HCl when soluble chlorocompex is formed:
Pb + 4HCl(conc) = H2[PbCl4] + H2
SnCl2 + HCl = H[SnCl3]
Ge and Sn dissolve in concentrated H2SO4:
Ge(Sn) + 4H2SO4(conc) = Ge(Sn)(SO4)2 + 2SO2 + 4H2O
This reaction does not virtually proceed with Pb due to low solubility of PbSO4. Although when H2SO4 concentration exceeds 80%, the reaction proceeds well and soluble acid salt Pb(HSO4)2 or complex acid H2[Pb(SO4)2] is formed:
Pb + 3H2SO4(conc.) = Pb(HSO4)2 + SO2 + 2H2O
Reactions with HNO3:
Ge + 4HNO3(conc.) = H2GeO3 + 4NO2 + H2O (germanic acid)
4Sn + 10HNO3(very dil., 3%) = 4Sn(NO3)2 + NH4NO3 + 3H2O
3Sn + 4HNO3(dil., 30%) = 3SnO2 + 4NO + 2H2O [(SnO2)x(H2O)y - b -stannic acid]
Sn + 4HNO3(conc., >60%) = SnO2 + 4NO2 + 2H2O
Pb + 4HNO3(conc.) = Pb(NO3)2 + 2NO2 + 2H2O
Concentrated HNO3 passivates Pb, since Pb(NO3)2 is insoluble in concentrated HNO3, although it dissolves well in water, that is why Pb reacts with diluted HNO3 actively:
3Pb + 8HNO3(dil.) = 3Pb(NO3)2 + 2NO + 4H2O
[Conclusion: Sn occupies an intermediate position: it reacts with concentrated HNO3 like Ge, and with diluted as Pb].
Formation of octahedral complexes of these elements is possible as a result of d2sp3-hybridization.
Ge and Sn dissolve well in silicic (HF+HNO3) and simple (HCl+HNO3) aqua regua forming H2 [XF6] and H2 [XCl6] (X = Ge, Sn):
3Ge + 4HNO3 + 18HF = 2 H2[GeF6] + 4NO + 8H2O
Interaction with alkalis: Ge has no interaction, but at the presence of oxidants:
Ge + 2NaOH + 2H2O2 = Na2[Ge(OH)6] (hexahydroxogermanate).
Sn and Pb dissolve slowly in strong alkalis when heated:
Sn + 2NaOH + 2H2O = Na2[Sn(OH)4] + H2
Pb + 2NaOH + 2H2O = Na2[Pb(OH)4] + H2
This interaction confirms the amphoteric nature of Pb, and, especially, Sn.
COMPOUNDS
Carbon
Carbides. They are binary compounds of carbon with more electropositive elements - metals, silicon, boron.
Carbides are formed only at high temperature interaction of carbon with elements (but technologically more frequent of carbon with oxides). All without exception they are very hard substances. Conventionally they can be divided into three large classes according the structure, character of bond and chemical properties:
· ionic – are formed by active metals (they are easily decomposed by water or acids);
· covalent – have an atomic crystalline lattice, are extraordinarily hard and chemically inert (SiC, B4C);
· inclusion are metal-like, are conductors of electric current, they are very hard and refractory, extraordinarily chemically inert. The atoms of carbon in them are located between the knots of crystalline lattice of metals. Their composition often does not answer the ordinary valence states of atoms of elements (Mn4C, Mn3C, Mn8C3; Cr3C2, Cr7C3; MoC, Mo2X, WC, W2C; VC; NbC; Nb2C; TaC; Ta2C). Sometimes they have no definite stoichiometry and belong to bertollides, for example, TiC0,6-1,0, VC0,58-1,0.
Ionic carbides. This name is only conditional. They are divided on 1) acetylenides (the most widespread class), 2) methanides and 3) those which are decomposed by acids with formation of mixture of hydrocarbons and hydrogen.
Acetylenides. Are formed by most active metals (for example, Li2C2), have in composition bivalent ion C22-. They are easily decomposed by water with exothermic heat effect:
СаС2 + 2Н2О = Са(ОН)2 + С2Н2 DН°298 = –125,5 kJ/mol
More frequently, worldwide is used and prepared CaC2 (~ 5 million of ton annually). It is used for preparation of C2H2 in gas welding and as reductant is in metallurgy. The carbide of calcium is preprec in electric furnaces at fusion of calcium oxide with coal:
СаО + 3С = СаС2 + СО DН°298 = 464,4 kJ/mol
Methanides. Are known only for the beryllium and aluminium (Be2C and Al4C3). With hot water or diluted acids they are decomposed with the formation of methane:
Al4C3 + 12H2O = 4Al(OH)3 + 3CH4
Carbides of the third type. These are Fe3C, Co3C and some other. Are decomposed by acids:
Fe3C + 6HCl = 3FeCl3 + CH4 + H2.
At the same time are formed other gaseous hydrocarbons.
To the separate type belongs carbide Mg2C3, which is formed from MgC2 subject to the condition of breaking of carbon off at 500°. At interaction with water, it gives pure propine:
Mg2C3 + H2O = 2Mg(OH)2 + CH3–CºCH
The covalent carbides. Most widespread are SiC, V4C. They are inorganic polymers, and widely used in industry. A carborundum SiC has high hardness and wearproof, chemically is very inert. V4C is very hard (~diamond) and chemically stable.
Carbides of inclusion . NbC and TaC are melted at 3500° and 3900° and are most refractory from all known substances. Carbides of tungsten and tantalum are extraordinarily hard and are used for creation of superhard alloys.
Such carbides are decomposed by oxidizing alkaline melts only:
2WC + 5O2 + 8NaOH 2Na2WO4 + 2Na2CO3 + 4H2O
WC is oxidized in this reaction. In this case, it is possible conditionally to adopt the oxidation states of W and C equal to zero (0!). Then W gives 6 electrons, C - 4 electrons, and together WC gives 10 electrons to the molecule O2, which takes four electrons from WC. Basic coefficients are 2 before WC and 5 before O2.
HYDROGEN COMPOUNDS
Carbon
C—H bond energy makes up 416 kJ/mol and yields only to the bond C–F. These compounds are named hydrocarbons. Their quantity is approximately 5 million and they make the article of study of organic chemistry. We we will consider CH4 only.
Methane. CH4 - at ordinary conditions colourless gas, without a smell and taste. B.p. = -161°C, M.p. = -184 °C. Molecule it is comparative small and unpolar. For this reason it is heavily liquified and badly dissolved in water.
Production. There is no necessity in synthesis of CH4, although it can be synthesized. Reaction C with H2 though exothermic, but has a reversible character and at ordinary conditions does not occure:
С + Н2 Û СН4 DНо = -75,3 kJ/mol
Toward forming the CH4 reaction proceeds at heating of reagents in presence the crushed nickel catalyst at high pressures.
In laboratory. 1. heating of mixture CH3COOH with a natron lime:
CH3COOH + NaOH = Na2CO3 + CH4
2. hydrolysis of aluminium carbide:
Al4C3 + 12H2O = 4Al(OH)3 + 3CH4
Properties. CH4 easily gets ignited and burns with large emission of heat:
СН4 + 2О2 = СО2 + 2Н2О DН = -800 kJ.mol-1
Its mixture with air is explosive. It is visible from equation, that a mixture in which one volume of CH4 is mixed with two volumes of O2 is most explosive. Such correlation is easily achieved and from there follows a necessity to be extremely careful at use of natural gas. The mixture of methane with air at ordinary pressure ignites at ~ 700°C. Methane is toxic gas. For its exposure a little stinking mercaptane is added to natural gas.
CH4, as well as the other saturated hydrocarbons, at ordinary conditions is very inert. It does is not oxidized by such strong oxidants, as acidified solutions of KMnO4 K2Cr2O7 are. For inorganic chemistry are important the reactions of its partial oxidization:
CH4 + 1/2 О2 = СО + 2Н2 DН = -27.2 kJ/mol
and conversion:
СН4 + Н2О = СО + 3Н2 DН = 204.6 kJ/mol
In industry these reactions are used for preparation of hydrogen.
COMPOUNDS
Silicon
Silicon compounds
Si has oxidation state (+4) in compounds with non-metals; its oxidation state is (- 4) with the most active metals. Pure ionic compounds of this element are absent because a hypothetical Si +4 ion has enormous charge and small size that cannot be stabilized by any chemical environment. Therefore, it is very unstable.
Silicides of metals. Si can form binary compounds with metals. In the molten state, it interacts with Mg, Ca, Fe, Pt, Bi and etc. with the formation of silicides: Li3Si, CaSi, CaSi2 and etc.
Silicides of active metals are ionic-covalent compounds that decompose by water or acids:
4CaSi + 9H2O = CaSiO3 + 3SiH4 + 3Ca(OH)2
Silicon with d-metals forms metal-like, hard, and resistant to chemical attack substances of complex structure: Mn5Si3, Cr3Si, CrSi2 and etc.
Дата добавления: 2022-01-22; просмотров: 79; Мы поможем в написании вашей работы! |
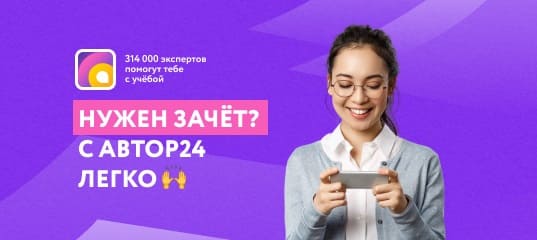
Мы поможем в написании ваших работ!