Environmental, economic and social implications of ocean warming.
Sergo Ordzhonikidze Russian State University for Geological Prospecting
The Ocean’s Role in the Hydrological Cycle
ВВ-17 Volojin Daniil
Москва 2018
The interactions between the seawater and freshwater segments of the hydrological cycle.
The global ocean covers 71 per cent of the Earth’s surface, and contains 97 per cent of all the surface water on Earth. Freshwater fluxes into the ocean include: direct runoff from continental rivers and lakes; seepage from groundwater; runoff, submarine melting and iceberg calving from the polar ice sheets; melting of sea ice; and direct precipitation that is mostly rainfall but also includes snowfall. Evaporation removes freshwater from the ocean. Of these processes, evaporation, precipitation and runoff are the most significant at the present time.
Using current best estimates, 85 per cent of surface evaporation and 77 per cent of surface rainfall occur over the oceans. Consequently, the ocean dominates the global hydrological cycle. Water leaving the ocean by evaporation condenses in the atmosphere and falls as precipitation, completing the cycle. Hydrological processes can also vary in time, and these temporal variations can manifest themselves as changes in global sea level if the net freshwater content of the ocean is altered. Precipitation results from the condensation of atmospheric water vapour, and is the single largest source of freshwater entering the ocean (~530,000 km 3/yr).
The source of water vapour is surface evaporation, which has a maximum over the subtropical oceans in the trade wind regions. The equatorward trade winds carry the water vapour evaporated in the subtropics to the Intertropical Convergence Zone near the equator, where the intense surface heating by the sun causes the warm moist air to rise, producing frequent convective thunderstorms and copious rain. The high rainfall and the high temperature support and affect life in the tropical rainforest.
Evaporation is enhanced as global mean temperature rises. The water-holding capacity of the atmosphere increases by 7 per cent for every degree Celsius of warming, as per the Clausius Clapeyron relationship. The increased atmospheric moisture content causes precipitation events to change in intensity, frequency, and duration and causes the global precipitation to increase by 2-3 percent for every degree Celsius of warming.
|
|
Direct runoff from the continents supplies about 40,000 km 3/yr of freshwater to the ocean. Runoff is the sum of all upstream sources of water, including continental precipitation, fluxes from lakes and aquifers, seasonal snow melt, and melting of mountain glaciers and ice caps. River discharge also carries a tremendous amount of solid sediments and dissolved nutrients to the continental shelves.
The polar ice sheets of Greenland and Antarctica are the largest reservoirs of freshwater on the planet, holding 7 m and 58 m of the sea-level equivalent, respectively. The net growth or shrinkage of such an ice sheet is a balance between the net accumulation of snow at the surface, the loss from meltwater runoff, and the calving of icebergs and submarine melting at tidewater margins, collectively known as marine ice loss. There is some debate about the relative importance of these in the case of Greenland. Van den Broeke et al. , show the volume transport to the ocean is almost evenly split between runoff of surface meltwater and marine ice loss. In a more recent work, Box and Colgan estimate marine ice loss at about twice the volume of meltwater (see Figure 5 in that article), with both marine ice loss and particularly runoff increasing rapidly since the late 1990s. According to the Arctic Monitoring and Assessment Programme, the annual mass of freshwater being added at the surface of the Greenland Ice Sheet (the surface mass balance) has decreased since 1990. Model re constructions suggest a 40% decrease from 350 Gt/y to 200 Gt/y in 2007. Accelerating ice discharge from outlet glaciers since 1995 - 2002 is widespread and has gradually moved further northward along the west coast of Greenland with global warming. According to AMAP, the ice discharge has increased from the pre-1990 value of 300 Gt/y to 400 Gt/y in 2005.
Antarctica’s climate is much colder, hence surface meltwater contributions are negligible and mass loss is dominated by submarine melting and ice flow across the grounding line where this ice meets the ocean floor. Freshwater fluxes from ice sheets differ from continental river runoff in two important respects. First, large fractions of both Antarctic ice sheets are grounded well below sea level in deep fjords or continental shelf embayments; therefore freshwater is injected not at the surface of the ocean but at several hundred meters water depth. This deep injection of freshwater enhances ocean stratification which, in turn, plays a role in ecosystem structure. Second, unlike rivers, which act as a point source for freshwater entering the ocean, icebergs calved at the grounding line constitute a distributed source of freshwater as they drift and melt in adjacent ocean basins.
|
|
Sea ice is one of the smallest reservoirs of freshwater by volume, but it exhibits enormous seasonal variability in spatial extent as it waxes and wanes over the polar oceans. By acting as a rigid cap, sea ice modulates the fluxes of heat, moisture and momentum between the atmosphere and the ocean. Summertime melting of Arctic sea ice is an important source of freshwater flux into the North Atlantic, and episodes of enhanced sea ice export to warmer latitudes farther south give rise to rapid freshening episodes, such as the Great Salinity Anomaly of the late 1960s. The spatial distributions of these freshwater fluxes drive important patterns in regional and global ocean circulation.
The Southern Ocean (defined as all ocean area south of 60°S) deserves special mention due to its role in the storage of heat (and carbon) for the entire planet. The Antarctic Circumpolar Current connects the three major southern ocean basins (South Atlantic, South Pacific and Indian) and is the largest current by volume in the world. The ACC flows eastward, circling the globe in a clockwise direction as viewed from the South Pole. In addition to providing a lateral connection between the major ocean basins (Atlantic, Indian, Pacific), the Southern Ocean also connects the shallow and deep parts of the ocean through a mechanism known as the meridional overturning circulation. Because of its capacity to bring deep water closer to the surface, and surface water to depths, the Southern Ocean forms an important pathway in the global transport of heat. Although there is no observational evidence at present, model studies indicate with a high degree of confidence that the Southern Ocean will become more stratified, weakening the surface-to-bottom connection that is the hallmark of present-day Southern Ocean circulation. A similar change is anticipated in the Arctic Ocean and subarctic seas, another region with this type of vertical connection between ocean levels. These changes will result in fresher, warmer surface ocean waters in the polar and subpolar regions, significantly altering their chemistry and ecosystems.
|
|
Imbalances in the freshwater cycle manifest themselves as changes in global sea level. Changes in global mean sea level are largely caused by a combination of changes in ocean heat content and exchanges of freshwater between the ocean and continents. When water is added to the ocean, global sea level adjusts, rapidly resulting in a relatively uniform spatial pattern for the seasonal ocean mass balance, as compared to the seasonal steric signal, which has very large regional amplitudes. ‘Steric’ refers to density changes in seawater due to changes in heat content and salinity. On annual scales, the maximum exchange of freshwater from land to ocean occurs in the late Northern Hemisphere summer, and therefore the seasonal ocean mass signal is in phase with total sea level with an amplitude of about 7 mm Because most of the ocean is in the Southern Hemisphere, the seasonal maximum in the steric component occurs in the late Southern Hemisphere summer, when heat storage in the majority of the ocean peaks. Because globally averaged sea level variations due to heat content changes largely cancel out between the Northern and Southern Hemispheres, the size of the steric signal, globally averaged, is only 4 mm.
Globally averaged sea level has risen at 3.2 mm/yr for the past two decades, of which about a third comes from thermal expansion. The remainder is due to fluxes of freshwater from the continents, which have increased as the melting of continental glaciers and ice sheets responds to higher temperatures. Multi-decadal fluctuations in equatorial and mid-latitude winds cause regional patterns in sea-level trends which are reflected in the Southern Oscillation and the Pacific decadal oscillation indices in the Pacific and northern Australia. Interannual changes in global mean sea level relative to the observed trend are largely linked to exchanges of water with the continents due to changes in precipitation patterns associated largely with the ENSO; this includes a drop of 5 mm during 2010-11 and rapid rebound in 2012-13.
|
|
Some key alterations are anticipated in the hydrological cycle due to global warming and climate change. Changes that have been identified include shifts in the seasonal distribution and amount of precipitation, an increase in extreme precipitation events, changes in the balance between snow and rain, accelerated melting of glacial ice, and of course sea-level rise. Although a global phenomenon, it is the impact of sea-level rise along the world’s coastlines that has major societal implications. The impacts of these changes are discussed in the next Section.
Changes in the rates of freshwater exchange between the ocean, atmosphere and continents have additional significant impacts. For example, spatial variations in the distribution of evaporation and precipitation create gradients in salinity and heat that in turn drive ocean circulation; ocean freshening also affects ecosystem structure.
Another factor potentially contributing to regional changes in the hydrological cycle are changes in ocean surface currents. For example, the warm surface temperatures of the large surface currents flowing at the western boundaries of the ocean basins provide significant amounts of heat and moisture to the atmosphere, with a profound impact on the regional hydrological cycle. Ocean surface currents like the seare forced by atmospheric winds and sensitive to changes in them - stronger winds can mean stronger currents and an intensification of their effects, as well as faster evaporation rates.Shifts in the location of winds can also alter these currents, for example causing the transport of anomalously warm waters. However, despite a well-documented increase in global wind speeds in the 1990s , the overall effect of climate change on winds is complex, and difficult to differentiate observationally from decadal-scale variability, and thus the ultimate effects of these currents on the hydrological cycle are difficult to predict with any high degree of confidence.
Environmental, economic and social implications of ocean warming.
As a consequence of changes in the hydrological cycle, increases in runoff, flooding, and sea-level rise are expected, and their potential impacts on society and natural environment are among the most serious issues confronting humankind.
The vulnerability of human systems to sea-level rise is strongly influenced by economic, social, political, environmental, institutional and cultural factors; such factors in turn will vary significantly in each specific region of the world, making quantification a challenging task. Three classes of vulnerability are identified:
1. Early impacts;
2. physically and economically vulnerable coastal communities; 2.
3. And physically vulnerable but economically "rich" coastalcommunities.
Outlines the main effects of relative sea-level rise on the natural system and provides examples of socio-economic system adaptations.
It is widely accepted that relative trends in sea-level rise pose a significant threat to coastal systems and low-lying areas around the world, due to inundation and erosion of coastlines and contamination of freshwater reserves and food crops; it is also likely that sea-level effects will be most pronounced during extreme episodes, such as coastal flooding arising from severe storm-induced surges, wave overtopping and rainfall runoff, and increases in sea level during ENSO events. An increase in global temperature of 4°C is anticipated to have significant socio-economic effects as sea-level rise, in combination with increasingly frequent severe storms, will displace populations. These processes will also place pressure on existing freshwater resources through saltwater contamination of the economy of many island nations is based on tourism; this too will be affected by sea-level rise through its direct effects on infrastructure and possibly also indirectly by the reduced availability of financial resources in the market.
Дата добавления: 2019-01-14; просмотров: 114; Мы поможем в написании вашей работы! |
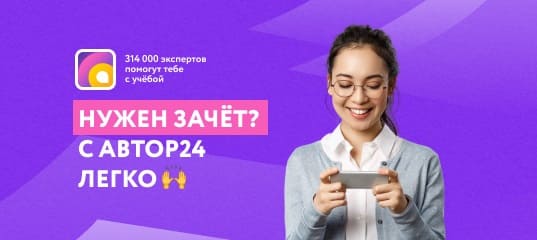
Мы поможем в написании ваших работ!