From the History of Holography
(D. Gabor’s lecture)
Holography is based on the wave nature of light, and this was demonstrated convincingly for the first time in 1801 by Thomas Young, by a wonderfully simple experiment. He let a ray of sunlight into a dark room, placed a dark screen in front of it pierced with two small pinholes, and beyond this, at some distance, a white screen. He then saw two darkish lines at both sides of a bright line, which gave him sufficient encouragement to repeat the experiment, this time with a spirit flame as light source, with a little salt in it, to produce the bright yellow sodium light. This time he saw a number of dark lines, regularly spaced; the first clear proof that light added to light can produce darkness. This phenomenon is called interference. Thomas Young had expected it because he believed in the wave theory of light. His great contribution to Christian Huygens’s original idea was the intuition that monochromatic light represents regular sinusoidal oscillations in a medium which at that time was called the “ether”. If this is so, it must be possible to produce more light by adding wave crest to wave crest, and darkness by adding wave crest to wave trough.
Light which is capable of interference is called “coherent”, and it is evident that in order to yield many interference fringes it must be very monochromatic. Coherence is conveniently measured by the path difference between two rays of the same source, by which they can differ while still giving observable interference contrast. This is called the coherence length, an important quantity in the theory and practice of holography; Lord Rayleigh and Albert Michelson were the first to understand that it is a reciprocal measure of the spectroscopic line width. Michelson used it for ingenious methods of spectral analysis and for the measurement of the diameter of stars.
Some Basic Concepts
Some of the questions about holography which come to mind immediately might serve as a good starting point for our discussion. They are: "What is a hologram? And how does holography work?" Note that the process is referred to as holography while the plate or film itself is referred to as a hologram. The terms holograms and holography were coined by Dennis Gabor (the father of holography) in 1947. The word hologram is derived from the Greek words "holos" meaning whole or complete and "gram" meaning message. Older English dictionaries define a hologram as a document (such as a last will and testament) handwritten by the person whose signature is attached.
|
|
The theory of holography was developed by Dennis Gabor, a Hungarian physicist, in the year 1947. His theory was originally intended to increase the resolving power of electron microscopes. Gabor proved his theory not with an electron beam, but with a light beam. The result was the first hologram ever made. The early holograms were legible, but plagued with many imperfections because Gabor did not have the correct light source to make crisp, clear holograms as we can today nor did he use the off axis reference beam which we will describe later. What was the light source he needed? The LASER, which was first made to operate in 1960.
Laser light differs drastically from all other light sources, man-made or natural, in one basic way which leads to several startling characteristics. Laser light can be coherent light. Ideally, this means that the light being emitted by the laser is of the same wavelength, and is in phase. These might be new terms for some of you, so let us form an analogy that might clarify the term coherence.
Let's say that you are flying over a freeway at rush hour, and directly below you is a long tunnel that all the cars must go through. Nothing is strange about the fact that all different styles and makes of motor vehicles emerge from the tunnel at differing velocities. A Cadillac at 75 mph, a Volkswagen at 45 mph, a motorcycle at 60 mph, a truck at 40. The distances between vehicles also vary. Thus you have different types of vehicles at varying speeds, and at constantly changing distances between each other.
But then something vary strange takes place; you see that more and more 1973 Cadillac Coupe de Villes are emerging. No, wait, look! All the cars coming out of the tunnel are 1973 Cadillac Coupe de Villes, gold with tinted windows, exactly alike, (a situation not totally uncommon in some carefully chosen Southern California suburbs). Not only are they the same year, make and color, but they are all traveling at exactly the same speed and all bumper to bumper, never changing. So that if you just happened to have a stopwatch to hand you would find that the cars are exiting at a rate of one car per second. If you were to leave, or more likely, pass out from the fumes, you would observe upon reawakening that the exit rate of the cars is still exactly one car per second. The cars are in phase.
|
|
The way in which coherent light is emitted from a laser is analogous. Keep in mind that although absolute 100% coherence is rarely, if ever, attained, there are certain types of lasers readily available which have sufficient coherent to make excellent off axis holograms. The light emitted from a laser is all exactly the same type, or make, depending upon the characteristics of the substance which is lasing. I will explain in the next chapter what the term laser means, and how the laser works to give coherent light. Right now it is important to remember that the frequency of laser light is unvarying and that in the same medium, all light, i.e., light of different wavelengths of frequency, travels at the same speed.
It's true that all electromagnetic radiation, including the very small portion we call visible light, travels in a vacuum at the approximate finite speed of 186,000 miles per second. (Note, the velocity of light in a vacuum is one of nature's constants and is referred to by the letter c). Light waves, can oscillate at different frequencies and with correspondingly different wavelengths so that for any given amount of time, say one second, a greater number of shorter wavelengths of (blue) light would be emitted from a laser than longer vavelengths of (red) light. This does not mean that different wavelengths travel at different speeds. Again to the freeway analogy; given the same speed and same distance between cars, more Volkswagens (short wavelength) than Cadillacs (long wavelengths) would pass by a point in the same amount of time.
Now is a good time to define some terms used previously but that you will see throughout this explanation. Wavelength, usually symbolized by the the Greek letter for lambda, and frequency, symbolized by the Greek letter v pronounced nu, have a reciprocal relationship v = C. The shorter the wavelength, the higher the frequency and vice versa. The amplitude is the height or intensity of the wave. For example, a laser rated at 5mW (milliwatts, or thousandth of a watt) would give off light at the same frequency and wavelength as another laser of the same type rated at 1 mw. But the wave heights of the 5 mw laser light would be five times higher than that of the 1 mw laser.
|
|
The wavelength is the distance from one crest to the next; this is also one cycle. It seems logical that we would need some constant measure of time in order to count the cycles. This constant unit of time is usually one second. Thus the term cycles per second, or cps., which is often referred to as Hertz or Hz (in honor of the German Physicist Heinrich Rudolph Hertz, who discovered radio waves). The oscillation frequency of electromagnetic radiation in the visible region is approximately 10 Hz. Wavelengths of visible light are between 400 and 700 nanometers or billionths of a meter in length.
We have described light as energy that travels through space in a wave form. For our purposes in talking about holography this is the case. However, the theory of light has unfolded miraculously, involving such great minds as Issac Newton, Thomas Young, Christian Huygens, Max Planck, Niels Bohr and, of course, Albert Einstein. Still, the dual characterist of light remain one of the many puzzles of nature. The particlewave problem which we refer to was clarified somewhat in the year 1900 when Max Planck proposed that all electromagnetic energy is radiated in discrete packages which he called quanta, or singular quantum. Einstein later confirmed Plank's theory via the photoelectric effect and used the word photon to refer to these energy packages. Scientists today refer to light sometimes as particles (photons or quanta) and other times as continuous waves depending on the situation or experiment. The problem is not with nature but with our models or concepts of nature. It is always very important to remember not to let your idea or model of the way anything should be usurp the place in your mind of the way it is or might be. That place should always be open for new information whether it agrees with theory or not.
|
|
Light travels in a wave form. More precisely, a transverse wave form. The crests and troughs of the waves (which in the case of light are electromagnetic fields) are rising and falling in a direction at right angles to the direction of travel. A swell or wave in the ocean is a good example of transverse wave motion. You'll notice that the rising and falling action of the wave is at right angles to the direction of travel.
A simple proof of the wave theory was first demonstrated by an English physician named Thomas Young in 1802. He forced the light from a single light source to pass through a narrow slit and then forced that same light to pass through two more narrow slits placed within a fraction of an inch of each other. The light from the two slits fell on a screen. Surprisingly he saw not jut the simple accumulation of the light from both slits on the screen, but a pattern of light and dark lines. He believed the pattern
was the result of the mixing of the waves of light emanating from the respective slits.
At the time it was very difficult for the many justifiably avid fans of Issac Newton to incorporate this new discovery into Newton's particle theory of light. Newton tried to explain optical phenomena such as refraction and reflection in terms of gravitational-like effects. As it turned out later, in a way, Newton's theory was given partial confirmation by the Quantum Theory.
The lines or "fringes" which Young saw, we call the interference pattern of the two light waves. When a crest interferes with a crest it is positive, or constructive interference, resulting in a bright spot. On the other hand, when a crest meets a trough we have a dark area or destructive interference.
As mentioned earlier, light waves oscillate at approximately 10 Hz., or a million billion times per second. There is no machine known to man sensitive enough to record the individual fluctuations additive effect of the light waves of which at each second 10 to the power of 15 wavelengths are interacting on the screen. This number, like so many numbers you may encounter in such fields as physics, astronomy and electronics, is incomprehensible. Yet precise measurement is part and parcel of the advancement of science. Suffice it to say that one billion seconds, for example, equals roughly 30 years, and 10 to the power of 15 seconds is one millions times that.
If the number is so fantastic and if even today we can't measure the waves individually, how did we discover that light was electromagnetic radiation? It's all thanks to the amazingly successful mathematical theory of J. Clark Maxwell, developed in 1864. He predicted not only the electromagnetic nature of light but also the speed at which it travels. Einstein used these same equations as a basis for his theory of special relativity. However, in order to understand exactly how light waves are formed, we had to wait until the year 1900 and the aforementioned Quantum Theory.
(http://www.holography.ru/histeng.htm)
MODULE 10 LASER RANGEFINDING Texts A Pulsed Laser Rangefinding Systems B. C. Cooperative and Non-Cooperative Targets D. Atmospheric Aerosol Pollution Monitoring |
Terminology:
1) range – диапазон; расстояние;ranging – измерение расстояния, определение дальности, pulsed laser rangefinding system (rangefinder) – импульсный дальномер; modulated beam (modulated continuous wave) range-finder – фазовый дальномер; range resolution – разрешение по дальности;
2) heterodyne detection – гетеродинный прием;
3) sighting channel – визирный канал; sighting telescope – визирная труба; bore sighting (harmonization) – установка направления, юстировка;
4) transmitter (receiver) optical train – система оптических элементов передатчика
(приемника)
5) effective focal length – полезное (эквивалентное) фокусное расстояние;
6) energy – storage capacitor – емкостный конденсатор;
7) high-speed counter – высокоскоростной счётчик;
8) head amplifier – предусилитель;
9) threshold comparator – пороговое устройство (компаратор);
10) false alarm – ложная тревога;
11) clock frequency – тактовая частота;
12) first (last) pulse mode – измерение дальности по первому (последнему) импульсу;
13) low-impedance output – низкое выходное сопротивление.
Vocabulary Practice
1. Pick out the words that do not suit in the following groups:
a) radar, filter, receiver, amplifier, transmitter, lunar, rangefinder, resonator, splitter, detector;
b) distant, electronic, visible, coincident, circuit, focal, effective, radial, appreciable, additional;
c) accuracy, circuitry, equipment, detection, majority, source, precise, ratio, magnification, target.
2. Combine the words from groups A and В to make terms:
A B
1) electron | 7) last-pulse | a) alarm | g) ranging |
2) beam | 8) heterodyne | b) telescope | h) resolution |
3) false | 9) sighting | c) velocity | i) detection |
4) lunar | 10) focal | d) divergence | j) effect |
5) Doppler | 11) range | e) circuitry | k) laser |
6) Q~switched | 12) target radial | f) length | l) mode |
3. Choose the correct word to complete the sentence that follows:
a) pumping, pumped, pump
The overwhelming majority of laser rangefinders use an optically _______ solid-state laser as the source of transmitter power.
b) effectuate, effective, effectively, ineffective
The field of view of the receiver channel is determined by the ratio of the size of the detector to the ___________focal length of the receiver objective lens.
c) effect, affect, affected, affecting
The transmitted spot is often made 20 to 30% smaller than the receiver field of view so that small differences between the two optical axes do not ___________ the overall system accuracy.
d) future, farther, furthermore, further
The signal level is then ___________ increased in the main amplifier.
e} whether, weather, whatever, whereas
This technique allows the operator to choose ___________to display the first or the last range value received.
4. Match the terms on the left with those on the right:
1) precise lunar or satellite ranging | a) поле зрения приемного канала |
2) visible laser rangefinders | b) источники питания электронного оборудования |
3) the magnification of the transmitter telescope | c) лазерные дальномеры видимого диапазона |
4) the field of view of the receiver channel | d) точно измерение расстояния до Луны и спутников |
5) quartz-controlled oscillator | e) расстояние до требуемой цели |
6) threshold value | f) спектральный селективный фильтр |
7) storage register | g) кварцованный генератор |
8) the range of the wanted target | h) увеличение телескопа передатчика |
9) wavelength - selective filter | i) регистр памяти |
10) electronic supplies | j) пороговое значение |
READING AND DISCUSSION
TEXT 10A PULSED LASER RANGEFINDING SYSTEMS
Introduction
The overwhelming majority of laser rangefinders in operation at the present time use an optically pumped solid-state laser as the source of transmitter power, but pulsed CO2 laser systems are now also making an appearance. The principles upon which these systems work are common to all, and the differences between various instruments lie in the fine details of the optical design and of the electronic circuitry. Pulses of duration 10 to 50 ns (giving accuracies of a few metres) are usual, but shorter pulses may be used for precise lunar or satellite ranging, and longer pulses are used in heterodyne CO2 laser systems.
The Principal Optical Elements
Rangefinding systems generally have three optical channels; these are the sighting telescope, and the transmitter and receiver optical trains. It is normal practice, at least for visible or near-visible laser rangefinders, to combine two channels together in a single optical train and some designs even combine all three functions using polarization to separate the transmit and receive beams and a wavelength -selective filter to separate out the sighting channel.
The beam divergence produced by the laser resonator is decreased by the magnification of the transmitter telescope, and is usually in the region of 0.5 mrad for instruments operating in ranges up to 10 km. The field of view of the receiver channel is determined by the ratio of the size of the detector to the effective focal length of the receiver objective lens. The size of the transmitted beam and the image of the detector at the target are usually made to be about the same order of size although the transmitted spot is often made 20 to 30%smaller than the receiver field of view so that small differences between the two optical axes do not affect the overall system accuracy. The process of aligning the three optical axes of the system to be coincident is referred to as boresighting or harmonization.
Electronics
Upon acommand from the operator, the electronic supplies are turned on and the energy storage capacitor charged to its working voltage. Then, when the sighting telescope has been carefully aligned to point at the target, the transmitter pulse is initiated. Asmall fraction of the energy from the pulse is picked off and fed to a fast photodiode; the output from the diode initiates a high-speed counter which is clocked by pulses from a quartz-controlled oscillator. A small fraction of the energy reflected from the target is collected by the receiver lens which is focused on to the detector.
The output from the detector is fed to a head amplifier which converts the current developed by the detector in a high impedance to a low-impedance output while minimising the additional noise introduced by the conversion process. The signal level is then further increased in the main amplifier. The amplified signal is applied to a threshold comparator; if the signal exceeds an internally set voltage, the clock is stopped. The threshold has to be set so that noise signals alone only very rarely cross the threshold to create false alarms, and yet must not be set so high that there is an appreciable probability that true signals will be missed.
If the clock frequency is chosen correctly, the number of clock periods stored in the counter can be simply related to the range of the target. For example, if the clock frequency is set at 29.978 MHz, light will travel 10 m in one clock cycle and thus the range resolution of the instrument will be +-5. Some designs can cope with more than one pulse exceeding the threshold value; in this case the clock is not actually stopped by the signal crossing the threshold level but instead the clock count at each occurrence is fed into a set of storage registers, which are read out in sequence at the end of the ranging operation.
It is then left to the judgment of the operator to decide which is the true range to the target. A simpler variant of this technique allows the operator to choose whether to display the first or the last range value received. For example, when operating under conditions where smoke or haze ispresent it would be advantageous to use last-pulse mode, whereas when operating in clear conditions against a target outlined against a more distant hillside, the first pulse is most likely to correspond to the range of the wanted target.
4400 п.зн.
5. Questions to the text:
1) What types of lasers are mainly used in the present-day rangefinding systems as the source of transmitter power? 2) List the three optical channels of a typical rangefinding system.3) What is the function of a wavelength - selective filter?4) In what way can the field of view of the receiver channel be determined? 5) What is boresighting?6) List the principal circuit elements of a pulsed laser rangefinder?7) In what way does the threshold have to be set?8) When does the operator choose the last-pulse mode?
6. Speak about the optical elements of a pulsed laser rangefinder.
7. We distinguish two types of target: those giving deliberately enhanced return , called cooperative ( кооперируемые ) targets, and other targets, referred to as non-cooperative ( некооперируемые ). Read the texts given below and find out which of them deals with rangefinders using cooperative targets:
TEXT B
The optical depth of natural targets (trees, buildings and. vehicles) is in the range of a few metres. This sets a limit to the accuracy that is useful, and thus sets a lower limit to useful pulse length, of order 25 ns. This length of pulse fits well with those produced by Q-switched solid-state lasers or gain Q- switched gas lasers.
Even if the accuracy requirement were relaxed, there is an energy advantage in keeping to as short a pulse as the detector will handle. This may be readily seen for a receiver that detects individual photo-events (e.g. a photomultiplier). One is trying to distinguish a weak return from random counts due to noise, background, etc. Clearly if the photoevents from the return are concentrated into the smallest possible time interval, they will have a maximum statistical significance compared with background photoevents, especially when the probability of a background event in the time interval becomes very small. For a receiver incapable of detecting individual photoevents, a similar conclusion applies; for detecting weak returns it is advantageous to compress the available energy into the shortest pulse.
TEXT С
The prime aim is accuracy. The use of retroreflectors (уголковый отражатель) provides not only a precise target distance but makes signal strength a secondary problem (at least up to several kilometres range). Measurement speed is also a secondary consideration. The precision attainable in a phase measurement accurate to within 0.1% at 50 MHz (corresponding to 3 mm range uncertainty) corresponds to a timing accuracy of 20 ps, which would clearly be difficult to attain by direct measurement because detector response times are usually inadequate. The advantage in energy terms of long duration transmission for direct detection systems is, therefore, outweighed by the accuracy advantage attainable with modulated waveforms lasting many seconds.
2000 п.зн.
8. Read Text D and answer the following questions: 1) What technique is this text concerned with? 2) What are its practical applications? 3) What types of laser are usually employed? Why?
TEXT 10D ATMOSPHERIC AEROSOL POLLUTION MONITORING
By using a pulsed transmitter it is possible to obtain detailed range–resolved data on the distribution of atmospheric aerosols; this technique is generally referred to as lidar, from light detection and ranging by analogy with radar.
Equipments usually employ visible lasers, either ruby, frequency-doubled Nd, or dye lasers to take advantage of the larger scattering coefficients at shorter wavelengths and the high sensitivities of photomultiplier tubes. Since signals produced by scattering from the atmosphere are much less than those from solid targets, high transmitter powers and large receiver optics (250 to 500 mm diameter) are employed. Such systems can be used to study the distribution of pollution aerosols, the evolution of natural cloud formations, the effluent from smoke stacks, the tracking of insecticide clouds, etc. Returns from particles at altitudes as high as 15 to 20 km can be obtained. By making observations at a series of different elevations it is possible to build up a picture of the aerosol particle distribution around a single station at a given time. Alternatively, the temporal evolution of pollution can be studied by firing the laser beam vertically upwards and recording the variation of aerosol distribution as a function of time. This has been used, for example, to study the pollution build-up in the vicinity of motorways.
1400 п. зн.
9. Render the text given below in English. Use the following words and word-combinations: time-of-flight, Fizeau, Michelson, modulated – beam rangefinders, pre-laser days, to stem from, fast high-energy pulses, to revolutionize the capabilities:
Время прохождения оптического луча от источника до удаленной точки и обратно измерялось Физо, Майкельсоном и другими учеными в период с 1850 по 1930 г. с целью определения скорости света. В настоящее время измерение периода прохождения оптического луча лежит в основе целого класса оптических дальномеров, включающего фазовые дальномеры, которые появились до создания лазера, а также импульсные лазерные дальномеры, в основе которых лежат системы, впервые продемонстрированные в 1961 г. вскоре после создания лазера. Импульсные дальномеры, использующие некогерентные источники, были изучены до I960 г., но применение коротких высокоэнергетических импульсов и высоко-коллимированных монохроматических лучей, получение которых стало возможным с созданием лазеров с модулируемой добротностью, открыло новые огромные возможности. Позже сообщалось о появлении дальномеров, использующих С02 лазер с гетеродинным детектированием; эти дальномеры аналогичны микроволновым радиолокаторам и используют доплеровский эффект для нахождения радиальной скорости цели.
10. Determine the boundaries limiting words and sentences for the text to make sense:
ThetimeoffligthofanopticalbeamfromasoursetoadistantpointandbackwasmeasuredbyFizeauMichelsonandothersintheperiod1850to1930todeterminethevelocityoflightNowadaysthetimeofflightmeasurementisthebasisofaclassofopticalrangefindersthatincludesmodulatedbeamequipmentwithoriginsinprelaserdaysaswellasthepulsedlaserrangefindersthatstemfromthesystemsfirstdemonstratedin1961soonafterthediscoveryoflaserPulsedrangefindersusingincoherentsourceshadbeenstudiedbefore1960butthefasthighenergypulsesandhighlycollimatedmonochromaticbeamsavailablefromQswitchedlaserrevolutionizedthecapabilitiesMore recentlyC02laserrangefindingsystemsusingheterodynedetectionhavebeenreportedthesehavemanyanalogieswithmicrowaveradarsandusetheDopplereffecttogivetargetradialvelocity.
SUPPLEMENTARY READING TASKS
Spaceborne Laser Radar
Laser radar systems are being developed for various spaceborne applications. At present the greatest need for spaceborne radars is as an accurate guidance aide for spacecraft that are performing rendezvous and docking maneuvers. Precise knowledge of the relative position between two spacecraft performing a rendezvous maneuver is difficult and often impractical to obtain from ground - based radars and therefore accurate radars located on board the spacecraft are desirable. Also two spacecraft performing docking maneuvers require careful monitoring of closure rates and angular alignment, thus precision on-board radars can be a valuable aid in bringing them together safely. On-board radars can also be useful for continuous or periodic surveillance of small satellite spacecraft that are parked in orbits nearby their mother spacecraft. Laser radar systems are presently being considered for use on future spacecraft such as the NASA space shuttle and space station. Space shuttles can use laser radar to aid the transferring of cargo into and out of their payload bay. Laser radar can also be used to aid the rendezvous and docking of space shuttles with space stations and can allow space stations to monitor the location of small satellite spacecraft.
Lunar Laser Ranging Experiment
The Lunar Laser Ranging Experiment from the Apollo 11 mission
The ongoing Lunar Laser Ranging Experiment measures the distance between the Earth and the Moon using laser ranging. Lasers on Earth are aimed at retroreflectors previously planted on the Moon and the time delay for the reflected light to return is determined. The distance has been measured repeatedly over a period of more than 35 years.The experiment was first made possible by a retroreflector array installed on July 21, 1969, by the crew of the Apollo 11. Two more retroreflector arrays left by the Apollo 14 and Apollo 15 missions have contributed to the experiment. The unmanned Soviet Lunokhod 1 and Lunokhod 2 rovers carried smaller arrays. Reflected signals were initially received from Lunokhod 1, but no return signals have been detected since 1971, at least in part due to some uncertainty in its location on the Moon. Lunokhod 2's array continues to return signals to Earth.
Apollo 14 Lunar Ranging Retro Reflector (LRRR)
The Apollo 15 array is three times the size of the arrays left by the two earlier Apollo missions. Its size made it the target of three-quarters of the sample measurements taken in the first 25 years of the experiment. Improvements in technology since then have resulted in greater use of the smaller arrays, by sites such as the McDonald Observatory and the OCA Laser-Lune telemetry station affiliated with the Côte d'Azur Observatory. The work is progressing on increasing the accuracy of the Earth-Moon measurements to near millimeter accuracy.
(From Wikipedia, the free encyclopedia)
Apollo 11 Experiment Still Going Strong after 35 Years
It was the summer of '69. Director John Schlesinger's "Midnight Cowboy" had won the Oscar for Best Picture; the Rolling Stones' newly released "Honky Tonk Women" was climbing the charts; 400,000 people were gearing up to attend Woodstock…and America landed on the Moon, making "one giant leap for mankind."
On the afternoon of July 20, 1969, Apollo 11 astronauts Neil Armstrong and Edwin "Buzz" Aldrin explored the surface of the Moon for two and a half hours, collecting samples and taking photographs while Michael Collins orbited in the command module Columbia. On July 21, about an hour before the end of their final moonwalk, they left an experiment on the lunar surface which, after 35 years, continues to work as well as it did the day it got there. Called the lunar laser ranging experiment, it studies the Earth-Moon system and returns data to scientific centers around the world, including NASA's Jet Propulsion Laboratory.
"An accurate knowledge of the Moon's orbit and orientation is needed for future robotic and manned missions to our satellite," said Dr. James G. Williams, one of four JPL scientists who analyze the data from the Lunar Laser Ranging Experiment. "Scientists have been able to use the data they received through lunar laser ranging to study the Earth, the Moon and the character of gravity."
Scientists from various institutions who analyze the data from the lunar laser ranging experiment have observed, among other things, that the Moon is moving away from the Earth and has a fluid core, and that Einstein's Theory of Relativity is accurate.
The experiment consists of an instrument called the lunar laser ranging reflector, designed to reflect pulses of laser light fired from the Earth. The idea was to determine the round-trip travel time of a laser pulse from the Earth to the Moon and back again, thereby calculating the distance between the two bodies to unprecedented accuracy. Unlike the other scientific experiments left on the Moon, this reflector requires no power and is still functioning perfectly after 35 years.
The Apollo 11 laser reflector consists of 100 fused silica half cubes, called corner cubes, mounted in a 46-centimeter (18-inch) square aluminum panel. Each corner cube is 3.8 centimeters (1.5 inches) in diameter. Corner cubes reflect a beam of light directly back toward its point of origin; it is this fact that also makes them so useful in Earth surveying.
Three more reflectors have since been left on the Moon, including two by later Apollo 14 and 15 missions and one (built by the French) on the unmanned Soviet Lunokhod 2 rover. Each of the reflectors rests on the lunar surface in such a way that its flat face points toward the Earth.
The McDonald Observatory in Western Texas and a second observatory near the city of Grasse in southern France regularly send a laser beam through an optical telescope to hit one of the reflectors. The reflectors are too small to be seen from Earth, so even when the beam is correctly aligned in the telescope, actually hitting a lunar reflector is quite challenging. At the Moon's surface, the beam is a few kilometers or miles wide and scientists liken the task of properly aiming the beam to using a rifle to hit a moving dime 3.2 kilometers (two miles) away.
Once the laser beam hits a reflector, scientists at the observatories use sensitive filtering and amplification equipment to detect any return signal. The reflected light is too weak to be seen with the human eye, but under good conditions, one photon -- the fundamental particle of light -- will be received every few seconds.
The lunar laser ranging experiment is the only lunar investigation continuously operating since the Apollo project. Improvements in lasers and electronics over the years have led to measurements currently accurate to about 2 centimeters (less than one inch).
Scientists know the average distance between the centers of the Earth and the Moon is 385,000 kilometers (239,000 miles), implying that the modern lunar ranges have relative accuracies of better than one part in 10 billion. This level of accuracy represents one of the most precise distance measurements ever made and is equivalent to determining the distance between Los Angeles and New York to one hundredth of an inch. "Technical improvements at the observatories rejuvenate the lunar laser ranging effort," Williams said. "When the range accuracy improves, it is like getting a new experiment on the Moon."
To this end, a new lunar ranging instrument with significantly improved accuracy is being constructed at Apache Point Observatory in New Mexico by the University of California at San Diego and the University of Washington. "The usefulness of continued improvements in range determinations for further advancing our understanding of the Earth-Moon system will keep the lunar reflectors in service for years to come," Williams said.
(Jet Propulsion Laboratory, California Institute of Technology; http://www.jpl.nasa.gov/index.cfm)
Дата добавления: 2019-09-13; просмотров: 143; Мы поможем в написании вашей работы! |
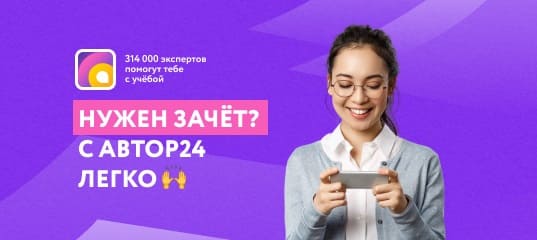
Мы поможем в написании ваших работ!